Flexible and stretchable fabric-based tactile sensor
Автор:Gereon Buscher, Risto Koiva, Carsten Schurmann, Robert Haschke and Helge J. Ritter
Abstract
In this extended abstract we introduce a novel fabric-based, flexible, and stretchable tactile sensor, capable of seamlessly covering natural shapes. Our developed sensor can have an arbitrary perimeter, can cover freeform surfaces and remains operational on top of soft padding such as a gel cushion, which is a prerequisite for building human-like, soft artificial palm and finger sensors. We discuss the construction of the sensor and evaluate its performance. The sensor is very robust and can withstand normal forces multiple magnitudes higher than what could be achieved by a human without sustaining damage.
I. INTRODUCTION
The importance of the sense of touch, especially for grasping and manipulations, has long been demonstrated by anesthetizing the fingertips of volunteers and thus deactivating their tactile receptors, resulting in failure to maintain a stable grasp [1]. The great majority of industrial robots manage to perform their tasks without tactile sensitivity due to almost perfect elimination of uncertainties in their operating environments. We argue that robots operating outside such heavily constrained environments will benefit strongly from force sensing capabilities. It has been shown that the manipulation of objects under nondeterministic conditions and handover tasks strongly benefits from the sense of touch [2]. Another immediate benefit is the possibility of increased safety by means of contact detection [3]. In our Manual Intelligence Lab [4], we use tactile sensors to measure the interaction forces at the human skin, allowing greater insight into manual interaction processes and indirectly facilitating the development of improved algorithms for robots.
II. FABRIC BASED TACTILE SENSOR
Humans and most visually appealing robots have curved body parts which limit the practical usage of traditional, rigid tactile sensors. Flexible tactile sensors have been proposed before, often based on flexible printed circuit boards [5]–[7]. The film-like substrate can be flexed in one dimension at a time. With a clever design that cuts the film carrier, tactile sensors capable of covering two dimensional curvatures have been demonstrated [8]–[10].
Stretchable materials can adapt to arbitrary, even dynamically changing surfaces much better. We present a flexible and stretchable tactile sensor based on layers of carefully selected fabrics, resulting in a very robust sensor, with a thickness of ≈1.5mm that can be cut and sewn in the same way as a common fabric, thus allowing a wide variety of final shapes to be produced. After evaluating numerous compositions of various conductive fabrics, we settled for a design using 4 layers of different plain and conductive fabrics ensuring good elasticity of the compound sensor [Fig. 1]. The sensor is based on the piezoresistive effect, where the electrical resistance of a material changes under mechanical pressure. Our sensor uses a piezoelectric, stretchable knitted fabric, where the individual fibers within the fabric are coated on a nano-scale with inherently-conductive polymers. The material resistance is determined by the thickness of the applied coating.
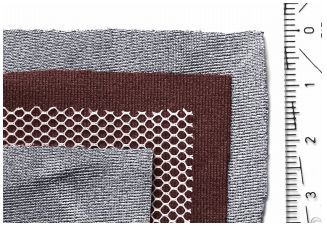
Fig. 1. The 4 fabric layers, as embedded in the flexible tactile sensor. A piezoresistive fabric and honeycomb mesh are located between the two highly conductive electrode layers
By placing the piezoresistive fabric between two highly conductive materials, we can observe a change in the resistance measured at the two outer layers when pressure is applied to the compound. These outer layers constitute the low impedance electrodes for transporting current into and out of the sensor with minimal losses. The low impedance of less than 2Ω per square specimen is achieved by plating nylon knitted fabric with pure silver particles.
During our experiments we discovered higher signal repeatability, especially in the subtle force range (0 to 5N), with the introduction of an additional nonconductive meshed layer between the middle piezoresistive and one of the electrode layers. Sensor sensitivity was found to depend on the thickness of the meshed layer and on the size of the mesh openings, with larger openings and thinner layers producing better sensitivity to first touch (determined by the smallest detectable force). With this additional mesh layer, the sensor has a very high matching concave probe with 3 stretch levels: 15% (red), 5% (blue) and unstretched sensor (green datapoints), while loading from idle to 35N. All measurements were repeated 10 times. resistivity (in the range of GΩ for a 50x50mm sample) when not acted upon. After establishing contact between the electrode and the piezoresistive sensor material (≥0.1N required), the single tactile sensor cell operates as a parallel circuit of force sensitive resistors. The high resistance in its idle state has the additional benefit of minimizing the current flow through the sensor and thus minimizing energy loss. This ensures a longer runtime of battery powered portable systems and simultaneously has the positive side-effect of significantly reducing the heat produced by the sensor. Especially in large-scale applications, such as covering the floor of a room, this becomes an important feature.
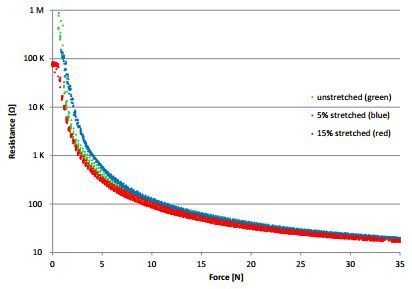
Fig. 2. The sensor resistance as measured on top of an 80mm radius convex surface using 3cm 2 matching concave probe with 3 stretch levels: 15% (red), 5% (blue) and unstretched sensor (green datapoints), while loading from idle to 35N. All measurements were repeated 10 times.
III. SENSOR EVALUATION
The composite 4-layer sensor remains stretchable up to 25%, limited by the chosen mesh layer (the electrodes and the piezoresistive material alone remain stretchable up to 100%). To evaluate the performance of the tactile sensors, we use a custom built measurement bench with calibrated industrial strain gauge as a reference sensor. We gathered the sensor characteristics while stretched by bulging it over a convex plastic surface with 80mm radius using numerous stretch ratios and by loading the sensor from idle to 35N. Approximately 160 data points were gathered in a single trial, lasting approximately 2 minutes. Fig. 2 depicts the sensor output over 10 consecutive trials at 3 stretch ratios. Starting from ≈10% stretch ratio, the idle resistivity decreases as the usually required force threshold has already been overcome. Apart from the first touch behavior, the sensor exhibits only marginal output differences between unstretched, 5% and 15% stretched states.
As can be seen from the quantitative results presented, the repeatability of the sensor is high. Flexibility, stretchability and simple construction result in a robust and universal tactile sensor that can capture force information from 3D surfaces.
IV. C ONCLUSIONS AND FUTURE WORK
Due to its inherent flexibility, the novel, thin, and stretch-able fabric-based tactile sensor is well suited for integration into almost arbitrary garments for humans or robots. As a consequence, wearable haptic sensing garments, such as shirts, trousers, caps or force sensitive datagloves can be built. Anthropomorphic robots can similarly be covered and given a sense of touch throughout their entire body. The field of Ambient Intelligence can benefit from the fabric based tactile sensors such as ours by allowing the augmentation of rooms and furniture with tactile sensing and thus making them responsive to the presence of people and pets. For example, sensitive bed-linen and pillows would allow less obtrusive monitoring of patients in hospitals. Furthermore, novel and more intuitive ways of interacting with computers and computer games using touch open up new possibilities.
As a next step, we will use the developed tactile sensor to augment the palm and fingers of the Shadow Robot Hands in our lab with the sense of touch. We also plan to develop an interactive, novel game interface incorporating the new sensor technology.
ACKNOWLEDGMENT
This work is supported by the DFG Center of Excellence EXC 277: Cognitive Interaction Technology (CITEC). We would like to thank Statex Production & Distribution plc. for providing fabric samples for this project.
REFERENCES
[1] G. Westling and R. S. Johansson, “Factors influencing the force control during precision grip,” Experimental Brain Research, vol. 53, pp. 277–284, 1984.
[2] J. Romano, K. Hsiao, G. Niemeyer, S. Chitta, and K. Kuchenbecker, “Human-inspired robotic grasp control with tactile sensing,” IEEE Transactions on Robotics, vol. 27, no. 6, pp. 1067 –1079, Dec. 2011.
[3] N. Elkmann, M. Fritzsche, and E. Schulenburg, “Tactile sensing for safe physical human-robot interaction,” in The 4th International Conference on Advances in Computer-Human Interactions (AHCI 2011), Feb. 2011, pp. 212–217.
[4] J. Maycock, D. Dornbusch, C. Elbrechter, R. Haschke, T. Schack, and H. Ritter, “Approaching manual intelligence,” KI - K? unstliche Intelligenz, vol. 24, pp. 287–294, 2010. [Online]. Available: http: //dx.doi.org/10.1007/s13218-010-0064-9
[5] O. Kerpa, K. Weiss, and H. Worn, “Development of a flexible tactile sensor system for a humanoid robot,” in Proceedings of the International Conference on Intelligent Robots and Systems (IROS 2003), vol. 1, Oct. 2003.
[6] M. Lowe, A. King, E. Lovett, and T. Papakostas, “Flexible tactile sensor technology: bringing haptics to life,” Sensor Review, vol. 24, no. 1, pp. 33–36, 2004.
[7] K. Kim, K. R. Lee, W. H. Kim, K.-B. Park, T.-H. Kim, J.-S. Kim, and J. J. Pak, “Polymer-based flexible tactile sensor up to 32*32 arrays integrated with interconnection terminals,” Sensors and Actuators A: Physical, vol. 156, no. 2, pp. 284 – 291, 2009. [Online]. Available:http://www.sciencedirect.com/science/article/pii/S092442470900377X
[8] Y. Ohmura, Y. Kuniyoshi, and A. Nagakubo, “Conformable and scalable tactile sensor skin for curved surfaces,” in IEEE International Confer-ence on Robotics and Automation, May 2006, pp. 1348 –1353.
[9] G. Cannata, M. Maggiali, G. Metta, and G. Sandini, “An embedded artificial skin for humanoid robots,” in IEEE International Conference on Multisensor Fusion and Integration for Intelligent Systems (MFI 2008), Aug. 2008, pp. 434 –438.
[10] T. Sagisaka, Y. Ohmura, Y. Kuniyoshi, A. Nagakubo, and K. Ozaki, “High-density conformable tactile sensing glove,” in IEEE-RAS Inter-national Conference on Humanoid Robots, Oct. 2011, pp. 537–542.