Improvement of environmentally sound technology rational use of thermal energy to the deep subsurface coal mines
Content
- Introduction
- 1. Relevance of the topic;
- 2. The purpose and objectives of research;
- 3. Sovrimennye ideas about the nature of geothermal energy and the ways of its use in deep coal mines:
- 3.1 Sources of geothermal energy classification of the International Energy Agency;
- 3.2 Features of distribution of low-grade geothermal energy ugnenosnoy thicker;
- 3.3 Removing low-grade heat subsurface using shaft geothermal heat exchangers;
- 4. Theoretical studies of regularities mutations both thermal conductivity of the rock mass;
- 5. Experimental studies of the marginal increase of thermal conductivity of rocks;
- 6. Improving the design of geothermal heat exchanger;
- References
1. Relevance of the topic
The most common types of fuel such as natural gas, oil will be exhausted in the near term, coal and uranium are also limited. Therefore, mankind is forced to switch to alternative forms of energy. Ecology in the mining regions explained intensive consumption of different types of energy produced from traditional types of fuels: natural gas, coal, oil, etc. This is accompanied by a huge greenhouse and toxic gases, aerosols and warmth. In this regard, the use of less harmful heat subsoil seems important environmental and resource task.
The analysis shows that the reserves of geothermal energy on the planet is sufficient to for a long time to meet the needs of all mankind, and its cost – one of the lowest among the renewable energy sources. However, suitable for use in modern power plants levels of warmth, with temperatures over 1500C, are at considerable depths, about 4 ... 5 thousand meters Basic modern ways to use geothermal energy based on downhole technologies. They are inherent disadvantages such as high cost of drilling operations; low productivity plants due to low capacity wells and high flow resistance fractured and porous reservoirs in the rocks; threat of erosion or clogging of heat-conducting channels in fractured rock massif, inaccessibility and the inability to monitor the work of the underground heat exchanger.
For mining regions of Ukraine, where there are no high-potential located shallow thermal resources, is a promising source of heat from the subsoil through the walls of mine workings in deep mines. If you implement this method in the space Broken geothermal energy can be concentrated in extended channels maze configuration. Using goafs underground mining – is the surest way to a lesser extent affected by many inherent disadvantages downhole technology. Is no need for survey and exploration work in the mine thermal environment known. When using exhaust openings reduced the cost of drilling wells and other works.
Scientists Donetsk National Technical University provides a method of extraction low potential energy coming from the bowels of the coolant and withdrawn from the walls of mine workings with mine using geothermal heat exchanger.
Mine geothermal heat exchanger (SHGT) – a set of mining, passed in a mountain range with a temperature of 30-50C rocks above, in which heat is extracted by heating the bowels moving into the workings of the coolant, usually air or water. Feature of the method is that for this purpose, developed area ie land massif, which is extracted from minerals, and their practical use is not envisaged in the long term. Under such conditions, the heat removal can be done hundreds of years, as the heat source will exist in the foreseeable future. Unlike well technologies in the process of heat transfer process is controlled by the threat or suffusion mudding - minimal. The duration of this type of installation is determined only by the stability of the space in which the heat transfer. Geothermal energy extraction efficiency will depend on the conditions of heat transfer from the depths of the array to the contour excavation.
In connection with the above, research on the mechanisms of a change in the thermal conductivity of the rock mass to improve the efficiency of extraction of geothermal energy and an improvement on the basis of this environmentally friendly technology rational use of thermal energy to the deep subsurface coal mines is an important scientific and technical challenge.
2. The purpose and objectives of research
Aim of this work is to improve the environmentally sound technology rational use of thermal energy to deep subsurface coal mines on the basis of the disclosure laws of change of thermal conductivity of the rock mass to improve the efficiency of extraction of geothermal energy, which would reduce the consumption of traditional energy resources.
To achieve this goal required the following tasks:
- Review current ideas about the nature of geothermal energy and the ways of its use in deep coal mines;
-To carry out theoretical studies patterns of change of thermal conductivity of the rock mass;
- Carry out pilot studies the marginal increase of thermal conductivity of rocks;
- To improve the design of the geothermal heat exchanger;
- Perform ecological and economic assessment of the proposed method of using low-grade geothermal energy for the needs of the enterprise and external customers.
Object of study – the distribution of low-grade geothermal energy undergoing influence of underground mining rock masses.
Subject of research – thermodynamic processes and conditions for the extraction of the rock mass and heat transfer from the walls of the coolant workings.
Research methods – when the master's work was the complex method: analysis and synthesis of information sources devoted to issues of geothermal energy; theoretical study of the process of heat transfer between the wall and the excavation coolant; theoretical and experimental studies of the design parameters of mine geothermal heat exchanger (SHGT); ecological and economic analysis of the use of advanced technologies for low-grade energy enterprises.
3 Modern ideas about the nature of geothermal energy and the ways of its use in deep coal mines
Geothermal energy – the energy of geothermal (geo – earth, thermal – thermal). The main source of this energy is a constant flow of heat from the hot interior directed to the ground. This heat is enough to melt the rocks beneath the crust, turning them into magma (which we sometimes see on the surface as lava). Most of the magma remains below ground and, like the furnace heats an upstream rock. When groundwater faced with this heat, they heat up - sometimes to a temperature of 371 ° C. In some places, especially at the edges of tectonic plates of the continents, as well as in so-called "hot spots" heat gets as close to the surface, it may be produced using geothermal wells.
3.1 Geothermal energy sources for the classification of the International Energy Agency:
• deposits of dry steam geothermal – relatively easy to develop, but are quite rare; however, half of the world's existing GeoTES these uses heat sources;
• sources of wet steam (a mixture of hot water and steam) – are more common, but their development has to address the issues of corrosion prevention equipment GeoTES and pollution (condensate removal due to the high degree of salinity);
• field of geothermal water (containing hot water or steam and water) – are so-called geothermal reservoirs, which are formed by filling the cavities underground water precipitation heated by magma close lying;
• hot dry rocks heated by magma (at a depth of 2 km or more) – they are the largest energy reserves;
• magma representing heated to 1300 ° C molten rock.
According to various estimates, the temperature in the center of the Earth is, at least, 66500S. The cooling rate of the Earth is approximately equal to 300-3500C in a billion years. Earth contains 42 • 1012 watts of heat, of which 2% is contained in the cortex and 98% – in the mantle and core. Modern technology can not provide the heat that is too deep, but 840000000000 W (2%) available geothermal energy can provide for the needs of mankind for a long time. Area around the edges of continental plates are the best place for the construction of geothermal power plants, because the crust in these areas is much thinner.
According to experts from all renewable energy is the lowest price for 1kW • h in Geothermal (табл.1.1).
Table 1.1 - Comparison of types of renewable energy.
Виды возобн. источников энергии | Установленная мощность (МВт) | Коифец. использов. мощности (%) | Стоимость 1кВт*ч сегодня (цент) | Стоимость 1кВт*ч в будущ (цент) | Стоимость 1кВт*ч установл . мощн.(тыс.дол.) | Доля выраб. энергии (%) | Прирост за последние 5 лет (%) |
Геотермальная | 10200 | 55 - 95 | 2 - 10 | 1 - 8 | 0,8 - 3,0 | 70,2 | 22 |
Ветер | 12500 | 20 - 30 | 5 - 13 | 3 - 10 | 1,1 - 1,7 | 27,1 | 30 |
Солнечная | 50 | 8 - 20 | 25 - 125 | 5 - 25 | 5 -10 | 2,1 | 30 |
Приливы | 34 | 20 - 30 | 8 - 15 | 8 - 15 | 1,7 - 2,5 | 0,6 | - |
3.2 Оsobennosti distribution of low-grade geothermal energy in coal-bearing strata
Development of mining method coalfields different coalfields are in a wide range of depths from 150 to 1300 ... 200m ... 1500m and deeper. Mines Republic of South Africa, Poland, the Czech Republic, Ukraine and some other countries have been mining in areas with temperatures of rock masses exceeding Tm = 35 ... 550C. This is much higher than physiologically tolerable to the human body the upper temperature limit is 25 ... 260C, that creates significant problems in ensuring a safe and healthy workplace climate.
The so-called geothermal zone (the distance from the recess by which to increase the temperature by one degree rocks) for many fields is 30 ... 33 m, while the gravitational temperature gradient is about 20 ... 400C immersion at 1000m.
In undisturbed mountain range where there is a thermostatic equilibrium, thermal energy (Q, J), coming from the depths, can be determined by the temperature difference in the rock layers with different isotherms:

where: S – area through which a heat flow, m2;
H – the difference between the depths of the isotherms, m;
p – density of the rock mass, kg/m3;
c – heat capacity of the rock mass, J / (kg * deg);
t – temperature difference between the isotherms deg.
The amount of energy is mainly determined by natural factors unchanged, only the flow area can be chosen arbitrarily. Therefore, most of the technology of geothermal heat are based on natural characteristics of the medium, when the first choice of places with high temperature gradient (the small difference between the depth of the isotherms).
The heat flux density is determined from the private:

where: Q – thermal energy, J.
S – the area through which a flow of thermal energy, m2;
τ – the duration of the heat flow with.
The energy flux density is inversely proportional to the square on which it is allocated and the duration of its validity. If we take into account that changes in planetary geothermal energy in the near future is not expected, the area from which the heat is removed, is the dominant factor.
In the case where the lead thickness at the breed mining operation, the thermal balance is not maintained. Violation of his due, periodic manifestation of additional heat sources: air pouring in streams; heated mass of transported mineral; heat from operating mining machines; water flows in pipes and gravity; exothermic oxidation of rocks and rotting organic matter; metabolic processes in organisms miners; phase transitions "liquid-gas"; geomehanichesih and some other processes. Prevail is the heat transfer between a mountain range and moving in mines airflow. In active mines air temperature should not be higher than the permissible maximum physiological safety rules - TFM = 25 ... 260C. At deep levels, where the temperature of the rock mass Tm> TFM provide or decrease in temperature through the use of different ventilation methods: intensification of ventilation, dilution flow of heated air cool, use air conditioning plants.
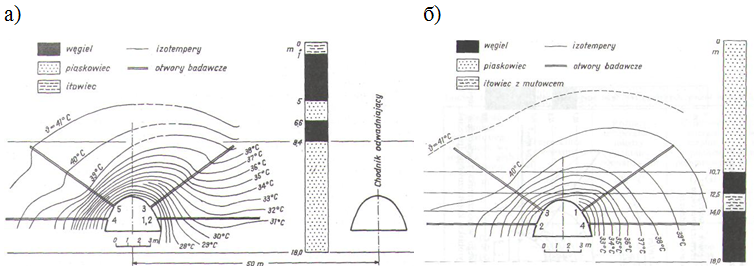
Figure 1.4 – Temperature fields in the rocks surrounding the field (s) and edge (b) 1030m horizon drifts in the mine "Halemba": 1,2 ... 5 – Room temperature wells for research.

where: λ – thermal conductivity, W / (m • deg);
Dt-temperature difference between the isotherms, hail;
l – distance between two energy levels, m
In this case, the smoothing effect of several factors, and it becomes clear that the density of heat flow is distributed fairly symmetrically relative to the vertical plane passing through the axis of generation (Figure 1.5).
Heat flux density increases as the contour generation from the depths of the array, although the absolute amount of energy – decreases due to the decrease in temperature. The data and observations of other researchers show that under the influence of mining and geological factors reduced the level of thermal energy transferred rock mass in the cross section of excavation and the marginal array.
Nevertheless, a number of researchers claims based on observations of mine that when a sufficiently long route traffic flow ventilation, the temperature condition persists for several years and even decades. This suggests that there are conditions when set dynamically equilibrium temperature field, and the extraction of energy from it can be done indefinitely.
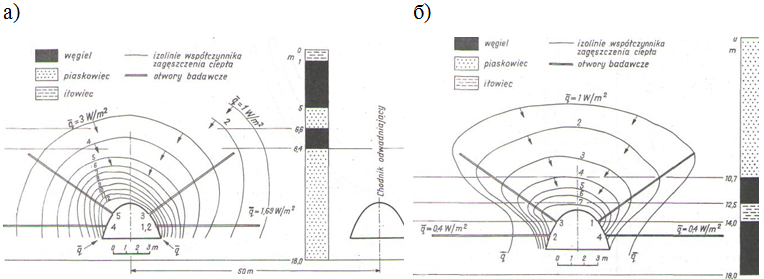
Figure 1.5 – Distribution of the heat flux density (q, W \ m2) in the surrounding mine workings (see Figure 1.4) rocks.
In tectonically quiet areas in the absence of steam hydrotherms and deep-seated hot rocks mine energy use is currently the most promising low potential inexhaustible source of clean energy.
3.3 Removing low-grade heat subsurface using shaft geothermal heat exchangers
The current situation in the Ukrainian coal industry crisis situation due to both organizational and technical factors so natural. For almost two centuries during the development of the Donbass coal deposits occurring at relatively shallow depths, powerful coal seams are exhausted. Development of medium and small power reservoirs have to be carried out in the field of coal containing methane array at temperatures relatively unstable country rocks more than 30 ... 500C. Coal mining is associated with irreversible negative consequences of ecological character: salinity of surface water and groundwater; emissions of greenhouse and radioactive gases and aerosols; deformations and seizure under the surface of the blades. This has resulted in the deterioration of mine safety and the rising cost of coal mined. Condition mine fund and existing technologies, along with organizational and economic problems do not allow significantly alter this trend. One of the possible ways to overcome the crisis - the use of a qualitatively new technological approaches to the development of underground deposits.
One of the clean energy sources can be subsoil heat extracted from the mining companies. The basis for this proposal was the results of long-term observations of air temperature, ventilation moving on long routes. Domestic and foreign researchers have found that the air passing a few kilometers of the underground mine workings, can be heated to the temperature of the array. Such thermal regime remains constant for decades, fueled by the flow of heat coming from the lower layers of the mantle of the planet. DNTU scientists found that the increase of energy can be extracted, creating waste in parts of the mountain range, the so-called geothermal heat exchangers. In the future, it is possible to use for deep mines of geothermal energy for technological purposes and for sale to customers in the form of electricity and heat, other types of products.
Mine geothermal heat exchanger (SHGT) - a set of mining, passed in a mountain range with a temperature of rocks TM ~ 30 ... 50 ° C and above, in which heat is extracted by heating the bowels moving into the workings of the coolant, usually air or water. Network Configuration channels can have a wide variety of circuit: series, parallel, and combined.
We can distinguish several characteristic modes of heat exchangers. In driving workings – construction mode (MS) when exposed surface generation air-cooled temperature array (TM) to airflow temperature (Tg) (Fig. 2.1). The duration of this state (tc) is determined by the pace of development and equipment SHGT. When operating as a production SHGT main mode used "discharge" the battery to heat or operating mode (PP), the massif around the canals is cooled by heat exchange with the air flow from the array temperature (Tm) to some admissible (Tg) for the period (tp). This is preceded by a period of "standing:" svezheproydennoy generation, ie restoration of the original temperature field of the array (cpi) to (TM) or preparative regimen (PR), duration (ts). Such a period of existence SHGT required to restore the temperature of (Tg) to (TM) for the estimated time (td) - The "charging" the battery heat (RH), the chilled zone array warmed by the heat leakage from the bowels.
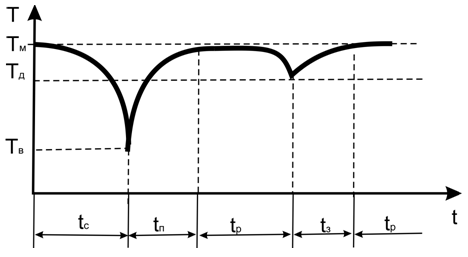
Figure 2.1 - Change of temperature (T) on the channel surface mine geothermal heat exchanger for the periods of construction (tc); provisioning (ts); work (tp); Charging (td): Tm, Tv, Tg - temperature, respectively, of the array of coolant (air), the allowable cooling channel walls.
4 Theoretical studies of patterns of change of thermal conductivity of the rock mass
Running time SHGT in operational mode is defined by the incoming and withdrawn from the bowels of the heated heat carrier. In the case where the amount of heat carried away is less than or equal to the number entering the heat exchanger can operate indefinitely in an operational mode. However, the task of ensuring maximum number of consumers of energy is achieved by increasing the coolant flow SHGT that entails a reduction of the period of his work in TT.
Intensive cooling heat exchanger walls reduces the temperature in the host rock mass production mountain. Ensuring constant heat of the rock massif near channel heat exchanger is only possible due to the inflow of heat bowels. From classical physics it is known that the amount of heat transmitted to the substance layer thickness Δl area S, while maintaining its temperature difference ΔT planes at time t is determined from the expression:
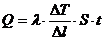
where: λ–thermal conductivity material, W / (m °).
Characteristic of the conductivity of the medium is the coefficient λ. Limit changes in this indicator for sedimentary rocks is 0,2 <λ <12, including sandstone 1,3 <λ <4,2, limestone 1,0 <λ <2,3, mudstone 1,5 <λ < 2.2. In layered rocks observed higher value of the thermal conductivity along the stratification compared to the measured perpendicular to the layering. Coefficient of thermal conductivity anisotropy of layered rocks is an average of 1.1 ... 1.5.
These indicators are inherent in continuous unbroken cracked rock massif. In the case where the medium is divided by cracks, the rate decreases by about two orders of magnitude, since the crack is situated between the air (λ = 0,026), or another gas, with approximately the same level of conductivity. Heat transport in fractured arrays occurs through point contacts breed and gas cavities crack fillers. Intensity of the process is determined by the area ratio of the contacting surfaces and the separated gas. Near the contour generation fracture has a value greater than the array, since under the action of rock pressure in the marginal area of ??rock collapse, and increases the number of cracks per unit area, as well as the width of their disclosure, ie thickness of the gas layer through which heat transfer. In pristine mountain works by an array of cracks is much smaller width of disclosure is low, and therefore the thermal conductivity much more. In addition, the gas-bearing media, which include coal-bearing rock strata, adsorbed on solid surfaces polymolecular condensed gas layers provide increased relatively free gas thermal conductivity. Therefore, microcracks and micropores opening width which is less than the mean free path of the gas molecules can be represented as healed cavity with increased value of λ.
Extremely important role played by the shape and orientation of the cavities in rocks. If they are like plates (cracks) is the thermal conductivity is significantly reduced when the location of cavities perpendicular to heat flow. Macrocracks deployed mainly in the field of geological faults and arrays in zones of influence on the workings enclosing rocks. So around the contour of development working under the action of rock pressure area formed destruction of rocks separated Macrocracks.
Studies have found that in the process of maintaining and further preparatory workings in their sides is the development of the existing array of microcracks and their transition into the category of macro-cracks. Simultaneously develop macrocracks technological system, oriented parallel to the contour of the pedigree in the radial direction to the axis of generation. Having this kind of rock discontinuities leads to drastic deterioration of conductivity of the medium.
Integral indicator of the overall fracture environment, assumed rate of fracture Kmn emptiness, which shows how much of the volume of rock fractures constitute cavity. Schematic representation of the change in the nature of KTP in the sides of mine workings, with respect to the question of the origin of endogenous fires is shown in Figure 3.12.
In a well-developed array of pristine consisting of multiple systems network of microcracks, as this array corresponds to a coefficient of fracture Ktp0 emptiness. During excavation the formation of new microcracks existing disclosure and their transition into the category of macro-cracks. Crack volume emptiness exponentially decreases depending on the contour generation in depth array. Near the contour generation volume macrocracks prevails over the volume of microcracks (Lmin). With the removal of deep array volume increases microcracks, and at a certain distance (Lmax), corresponding to the zone of influence of mining operations, the volume will macrocracks disproportionately small compared to the volume of microcracks.
These data show that the presence of fracture can significantly reduce the thermal conductivity of the rock mass, and thus decrease power silo geothermal heat exchanger. For practical calculations for values ??of porosity (P) is not more than 20% is proposed to use a cubic dependence of the type:
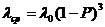
The porosity of the rock mass can be changed 0.03 <P <0.2, according to the expression 3.33 coefficient of thermal conductivity will be - 0,91 <λ0 <0,512. Fracture orientation parallel to the contour excavation determines the decrease heat flow is in the direction of the development of the cavity, which is contrary to our aspirations.
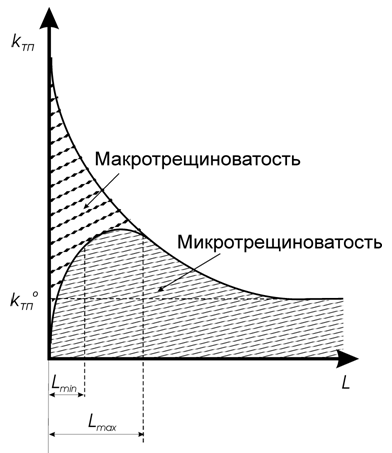
Figure 3.12 - Diagram of distribution coefficient fracture emptiness (KTP) in the sides of excavation: Ktpo - background level of fracture emptiness intact array, Lmax, L min - the boundary of the maximal and minimal development makrotreschinovatosti.
Significantly increases the value of the coefficient of thermal conductivity λ presence in rocks water. In liquid state, it may be contained in the rocks in the form of films, and in free form. Filling the pores and cavities in the case of saturation of rocks, water may improve the thermal conductivity is higher than the arrays characteristic of dry solid sample. Gravitational water contained in the underlying preparatory development of fractured rocks. Furthermore, because of the high air humidity silo (relative humidity greater than 80%) in the open pores and cracks attaching hygroscopic moisture.
The presence of water flowing on the ground working, improves heat transfer and increase the capacity of the heat flux transmitted from the depths of the coolant. Calculations show rocks with a significant amount of porosity and high humidity, characterized by a quantity approximately twice larger than that of dry samples fractured rocks (Fig. 3.13).
The problem of the artificial increase of the thermal conductivity of fractured rocks. This might be the case when open macrocracks fill able to solidify the composition (ie, reduce the porosity of the medium) having more than water. Possesses such characteristics, for example, a suspension of bentonite clay 0.7 << 0.98. Especially increases the thermal conductivity of adding to the suspension of the fine graphite powder with a unique figure 116 << 174. Thus, forcing the suspension of bentonite clay with graphite additives and surfactants for better wetting of the surface pores and cracks in the surrounding array production can reduce the negative impact of man-made and natural fractures and to improve the thermal performance SHGT. In this case, the thermal conductivity of rocks processed increases significantly, and correspondingly increased power SHGT. Thus regulation of the thermal properties of the array can be implemented in a very wide range.
5 Experimental studies of the marginal increase of thermal conductivity of rocks
By experiment was installed and proved the possibility of using a mixture of clay and graphite as a thermally conductive composition. For the experiment, 40 samples were prepared with various concentrations of graphite powder (0%, 5%, 10%, 15%, 20%, 50%, 75% and 100%). Sample preparation is carried out in the laboratory using a press. Making direct measurements carried out in accordance with GOST 25493-82. In addition, measurements were made of the thermal conductivity of clay-graphite mixture moistened. For this, the samples were placed in a desiccator over water surface and held there until the maximum degree of saturation, which is determined from the weight increase of the sample. The resulting thermal conductivities hydrated alumina graphite mixtures are presented in Table. 1.
Table 1 - Effect of graphite content on the coefficient of thermal conductivity of dry and hydrated alumina graphite mixture.
Содержание графита глино–графитной смеси, Сгр, %вес. | Коэфициент теплопроводимости сухой глино–графитной смеси, Дж | Коэфициент теплопроводимости увлажненной глино–графитной смеси, Дж | Увеличение коэфициента теплопроводимости сухойглино–графитной смеси, % | Увеличение коэфициента теплопроводимости увлажненной глино–графитной смеси, Д% |
0 | 4,87 | 6,18 | 0 | 0 |
5 | 5,1 | 6,7 | 4,72 | 8,4 |
10 | 5,7 | 7,25 | 17,0 | 17,3 |
15 | 6,69 | 7,66 | 37,4 | 23,9 |
20 | 7,69 | 8,41 | 57,9 | 36,08 |
50 | 10,17 | 15,89 | 108,83 | 157,12 |
75 | 11,9 | 12,57 | 144,35 | 130,40 |
100 | 15,57 | 13,68 | 219,71 | 121,36 |
Thus, the apparent effectiveness of the alumina-graphite mixture to increase the thermal conductivity. Reduction of the thermal conductivity with the concentration of the humidified sample graphite powder of 75% and 100% is due primarily hydrophobic property of the graphite powder.
Figure 2 shows plots of the thermal conductivity of dry and hydrated alumina graphite mixture of graphite powder concentration. The dependence of the thermal conductivity of dry clay-graphite mixture is described by the formula
y = 5,2945 e0, 0113h
bivariate correlation coefficient with the / r / = 0,95.
Dependence of the thermal conductivity hydrated alumina graphite mixture of graphite content is described by:
y = -0,0016 x2 0.2403 x 5.3171
bivariate correlation coefficient with the / r / = 0,87.
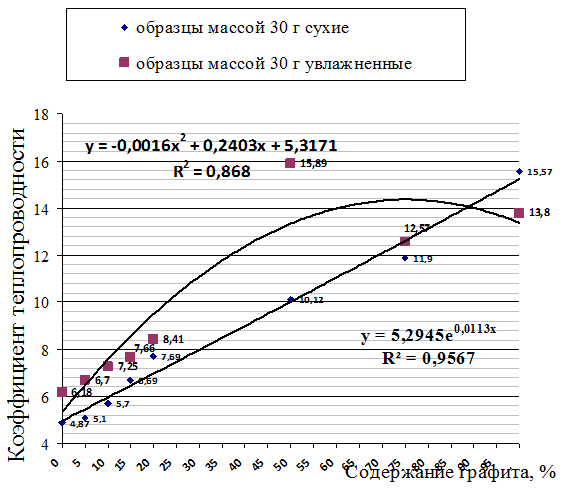
Figure 2 - The dependence of the thermal conductivity of the graphite content in the alumina-graphite mixture.
These tests and the results obtained according to suggest that there is an optimum value of the content in the alumina-graphite graphite mixture, wherein the thermal conductivity of the mixture in the wet state is maximized. For the experiment of wastes used in its optimum graphite content in the mixture is moistened with 75% wt. The results can be used to justify a thermally conductive filler of bolting, which will increase the efficiency of heat transfer from the rock massif coolant when extracting geothermal heat from the workings in deep mines.
6 Improving the design of geothermal heat exchanger
On the basis of experimental studies have proposed a method of anchoring, consisting of the following (Fig. 1). First analytically calculated depth zone of broken rocks lt, lz joint part and the hole in general lsh. Then the contour generation 1 in rockmass 2 holes drilled deep lsh diameter D2, larger than the diameter of the anchor 3 D1. Thereafter, holes are drilled to a depth of up to lt diameter D3, the diameter of the heat-conducting part of the hole should be at least twice the diameter of the anchor 3 D3 ≥ 2D1. Under this condition the growth of the heat flux through the "anchor - filler 'in the cavity generate the most significant.
Thereafter, holes are filled to the length ampoules joint part 7 and a resin fixative, using the contents of ampoules of the anchor 3 and thus stirred anchor is secured in a hole. At the same time preparing a thermally conductive filler 4 with high coefficient of thermal conductivity λt. As filler can be used a mixture of bentonite clay and powdered graphite. Dry pre-mixture ingredients are mixed in a 4:1 ratio by weight, that defines the desired value of the thermal conductivity. Then dry mixture shuts water to the consistency of thick slurry and pumped via slurry pumps in heat-conducting part of the drill holes.
From the production installation of the support washer provided between the wall 5 and generating one nut 6. For better heat transfer between the "anchor - filler" and making the air in the cavity 1, the support shaft 5 may be made of metal as a radiator - parts with the outer surface developed that increases the conductive heat transfer between the "anchor - filler" and mine cooled geothermal heat exchanger and promotes intensification process.
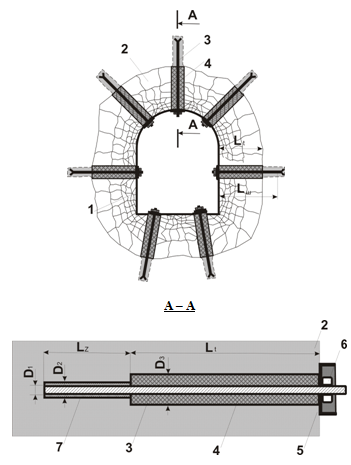
Figure 1 - The process of anchoring generation: 1 - preparatory development; 2 - rock mass; 3 - anchor; 4 - thermally conductive filler hole; 5 - support shaft; 6 - nut; 7 - fast setting; D1, D2, D3 - diameters, respectively, of the anchor hole in the joint portion of the hole in the heat-conducting portion; lsh, lz, lt - length, respectively, of the hole, locking and heat-conducting parts of the hole.
Interlocking the anchor portion 3 is thus outside the fractured region surrounding the formulation 1, i.e. at temperatures massif. Anchor 3 is a conductor of heat, intense spending to its low temperatures - in the cavity generation 1. Capacity heat flow is directly proportional to cross-sectional area master channel. However, in terms of saving metal anchors used irrationally large cross section. Therefore, the best use of combination devices that combine carrying rods of durable materials and the surrounding heat conductive elements having secondary importance as carriers, as well as providing increased thermal conductivity. After curing the mixture around the heat conducting anchors in boreholes three zones are formed with high thermal conductivity. Application of the method of fastening it possible to estimate the temperature increase in mining. Produced preparatory generation 1 Size = 5.0 m and H = 3.0 m was held at a depth of 1000 m in rocks with average strength R = 30 MPa using a tunnel boring machine. Host rocks were presented sandstone with an average coefficient of thermal conductivity λvp = 3.4 W / (m ° K), the temperature at 42 ° C was. Increasing the efficiency of heat transfer from the rock massif controlled temperature bearing washer 5. When attaching develop in the usual way, its temperature was 29oS. Drilled hole 4: № 1 - fixed in the usual way, № 2, 3, 4 - with the help of a heat-conducting diameter, respectively 36, 50, 72 mm
Capacity of the heat flow Q, J / c, transmitted through such anchoring systems, determined by the formula:

where delta T - temperature change of thrust washer, C;
lt - depth zone of broken rocks, m;
t - time to;
λa, λt - coefficients of thermal conductivity, respectively, anchor and thermally conductive filler 3 4, W / (m K);
D1, D3 - diameters, respectively, of the anchor in the hole 3 and the heat conducting part.
The most intense heat flux growth, and therefore the temperature was observed when the diameter of the hole in the heat-conducting portion D3 of not less than twice the diameter of the anchor body 3 D1.
The results of the experiment are summarized in the silo Table 1.
Table 1 - Changes in temperature bearing washer depending on the diameter of the hole with a thermally conductive filler.
№ шпура | D3, (мм) | Q/t, (Дж/с) | Тш, (С) | ΔТш, (С) | ΔQ/t, (Дж/с) | +/-, (%) |
1 | 36 (без теплопроводящего наполнителя) | 0,213 | 29 | - | - | - |
2 | 36 (с теплопроводящим наполнителем) | 0,225 | 31 | 2 | 0,-12 | +5,63 |
3 | 50 | 0,279 | 37 | 8 | 0,066 | +30,99 |
4 | 72 | 0,451 | 42 | 13 | 0,238 | +111,7 |
Thus, using the present method provides a method of anchoring improving the generation, wherein in the area by drilling boreholes broken rocks diameter not less than twice the diameter of the anchor, filling the gap between them and the heat conductive filler hole wall after installation and fixing of the anchor washer support having a surface branched heat exchange with the air output, provided the increase in the thermal conductivity of rocks enclosing the production of feed, which increases the efficiency of heat transfer from an array of rocks coolant.
References
1. Патент на корисну модель № 70012 Україна, МПК Е21D 13/00. Спосіб анкерного кріплення виробки/ В.К. Костенко, О.Л. Зав'ялова, С. Саліхерадж, О.С. Шипика; заявник і власник ДонНТУ. – № u201112926; заявл. 03.11.2011; опубл. 25.05.2012, Бюл. №10.
2. Патент на винахід №102020 Україна МПК E21D 21/00, E21D/00. «Спосіб анкерного кріплення виробки» Костенко В.К. . Зав'ялова О.Л., Салехирадж С. Шипика О.С., заявники і власники ДонНТУ. – № а 2011 12723; заявк.. 31.10.2011; публ., 27.05.2013 бюл. № 10.
3. Шипика А.С., Скринецкая И.В., Завьялова Е.Л. Использование геотермальной энергии в условиях глубоких шахт / Екологічні проблеми топливно-енергетичного комплексу: ІІІ регіональна наук. конф. аспірантів і студентів, 25 - 26 квітня 2012 р: зб. матер. конф. – Донецьк: ДонНТУ, 2012.- С.180-185.
4. Шипика А.С., Скринецкая И.В., Завьялова Е.Л. «Повышение эффективности использования теплопроводящих анкеров», «Комплексне використанння природних ресурсів»: V регіонал. конф., 6 грудня 2012 р.: зб. доповідей студентів та аспірантів. – Донецьк: ДонНТУ, 2012. - С.23-28.
5. С. Салехирадж, Шипика А. С., Завьялова Е.Л. Увеличение объема извлекаемой шахтным геотермальным теплообменником энергии выработок глубоких шахт/ «Комплексне використанння природних ресурсів»: IV регіонал. конф., 12 грудня 2011 р.: зб. доповідей студентів та аспірантів. – Донецьк: ДонНТУ, 2011. - С.28-31.
6. Завьялова Е.Л.Повышение эффективности использования теплопроводящих анкеров/ Е.Л. Завьялова, А.С. Шипика, И.В. Скринецкая// Вісті Донецького гірничого інституту. – Донецьк, 2012. – №1(30)-2(31) – С. 459 - 465.
7. Шипика А.С., Скринецкая И.В., Завьялова Е.Л. «Повышение эффективности использования теплопроводящих анкеров»/ Проблемы недропользования: Сборник научных трудов. Часть II / Национальный минерально-сырьевой университет «Горный»: VIII международный форум-конкурс молодых ученых: 24-26 апреля 2013 года. - Санкт-Петербург, 2013. - С. 198-200.