Abstract
Contents
- Introduction
- 1. Relevance of the topic
- 2. The purpose and objectives of the study, the planned results
- 3. Review of research and development
- 3.1 Overview of International Sources
- 3.2 Overview of local sources
- Conclusion
- References
Introduction
Daily all of us are necessary interacts with installations of artificial illumination. Therefore, we can confidently say that lighting devices are the most common among all existing engineering systems. The use of artificial lighting, of course, makes it possible to provide comfortable and productive activities of people in the absence of natural lighting, but has its own characteristics. Luminous flux of the light sources that receive AC voltage of the industrial frequency, characterized by pulsation, which can adversely affect the health of a person.
The main way to reduce the impact of such pulsations on human health is to control the parameter that characterizes it – the allowable ripple factor. Currently, the estimation of the pulsation of illumination is performed using the parameter mentioned above.
1. Relevance of the topic
As already mentioned above, lighting installations are the most common electrical devices among all existing and their influence most strongly affects the person. Therefore, we will consider in more detail one of the most powerful types of negative impact of lighting on man – rapid pulsations of the light flux. The luminous flux supplied with an AC voltage, pulsates with a double frequency, that is, when the lamp is powered by a voltage of the industrial frequency – the frequency of the oscillations of the illumination is 100 Hz.
This phenomenon also poses a serious danger in factories equipped with machines with open rotating parts that can appear stationary or rotating in the opposite direction in the case of pulsating illumination.
This phenomenon can be explained by the example of a stroboscopic top (Fig. 1–3), on the surface of which the radii are plotted at equal angles. If the displacement of the top on an angle equal to the angle between adjacent radii is equal to the period of pulsations or is an integer smaller than it, then the top appears to be stopped. With a slight increase in speed, the top will seem to have changed the direction of rotation. This phenomenon is known as the stroboscopic effect.
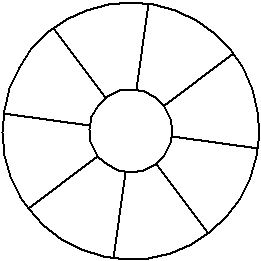
Figure 1 – Stroboscopic top. The frequency of rotation of the top is less than the frequency of pulsations
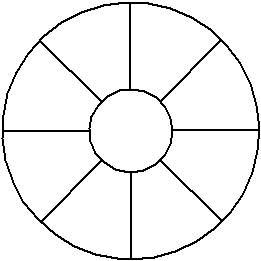
Figure 2 – Stroboscopic top. The frequency of rotation is equal to the frequency of pulsations
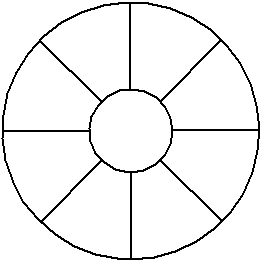
Figure 3 – Stroboscopic top. The frequency of rotation exceeds the frequency of pulsations
2. The purpose and objectives of the study, the planned results
The purpose of this project is to develop a dynamic model of electric light sources for analyzing pulsations of the light flux. To accomplish this task, you need to solve the following problems:
- To collect and evaluate information on the topic under consideration.
- Make a transition from the available indicator of the assessment of electromagnetic compatibility by pulsating the illumination to a universal indicator.
- Create an electronic database of the pulsations of illumination produced by various types of lamps.
- Make the transition from the existing dependence of labor productivity on the coefficient of ripple to the dependence of labor productivity on the universal indicator.
- Calculate doses of pulsations for different types of light sources.
- Calculate the decrease in labor productivity from the pulsation of illumination for various categories of visual work when using different lamps.
The scientific novelty of this work will be that, in contrast to the current practice of estimating pulsation by the ripple coefficient, a dynamic model of the pulsation estimate will be developed. It will take into account the shape of the graph of the process of changing the illumination, and not only its minimum and maximum ordinates.
3. Review of research and development
3.1 Overview of International sources
Recently, in 2002, D.Berson and his co-workers made a discovery of a new type of photoreceptor in the retina of mammals, including humans. It turned out that the function of reconfiguring the rhythms of daily activity under the influence of light is performed by some of the retinal ganglion cells (RGC), called light-sensitive cells. These cells react to light with wavelengths in the range 430-470 nm.[1]
They got the name ipRGC
(intrinsically photosensitive retinal ganglion cells). These studies on human and animal models began to clarify the neuroanatomy and neurophysiology of the photosensory system, which provides an input channel for circadian and neuropsychic regulation [2].
In this regard, the former president of ICI Professor V. Van Bommel expressed the idea of the need to develop new lighting criteria taking into account the new discovery. [3]
Also among other international sources describing the problem under consideration, it is worth mentioning the work of K. Pollack, L. Schmetterer and C. E. Riva under the title Influence of Flicker Frequency on Flicker-Induced Changes of Retinal Vessel Diameter
[5]. This work was published in the journal investigative ophthalmology and visual science
and is devoted to the study of the reaction of the human body to the effect of flickering light.
In his article, K. Pollack, L. Schmetterer and C. E. Riva studied the change in the diameter of the vessels of the retina of the human eye with the help of a special fundus camera designed for shooting the bottom of the eyeball.
To carry out the necessary measurements, they used a special installation – a retinal vascular analyzer, which included a fundus camera (поле 50°, model FF 450, Zeiss, Jena, Germany), videocamera, a real-time monitor and a computer with image analysis software for accurately determining the arterial and venous diameter of the retina.
Flicker was generated by focusing light from a 150W Xenon lamp system (Oriel, Stratford, CT) on a sector disk. A low-pass filter with a cut off at 550 nm was installed before the lamp (Laser Components, Olching, Germany). The light, passing through the disc, hit the fiber optic cable located behind the disc. The light at the output of the cable was inserted into the fundus lighting channel.
The study involved seven men and two women (mean age, 34 ± 11 years).
As a result, it was found that repeated stimulation with flickering light at a frequency of 8 Hz led to an average reaction in a venous diameter of 2.1%. Approximately 20 seconds after the start of the experiment, a stable change in the diameter of the vessels was usually observed. After the flicker ceased, a rapid decrease in diameter occurred, which reached the baseline in about 6 seconds. However, the measurements also showed that the diameter continued to fall below the baseline, reaching a minimum in about 10–40 seconds after the stimulus ceased. Scientists have yet to study the cause of this phenomenon.
Also, studies showed that during stimulation with flickering light, the diameter changed completely in all the vessels of the retina.
Based on the conducted experiments, it was concluded that the rate of blood in the arteries of the retina and veins will increase significantly, that can lead to the development of eye disorders. In addition, scientists came to the following conclusion: in cats and humans, the change in diameter will also depend on the brightness, wavelength and depth of modulation of the stimulus, as well as the average illumination of the retina and the location of the trunk.
The latter fact also indicates the relevance of this work and the need to develop a dynamic model for estimating the pulsations of the light flux.
3.2 Overview of local sources
Among the specialists of DonNTU, the problems of electromagnetic compatibility and the quality of lighting are handled by Doctor of Technical Sciences, Professor Kurenniy Eduard Grigorievich.
Among the publications devoted to the problem of calculating the dose of flicker, I can specify an article entitled Error in calculating doses of voltage flicker and checking the functioning of the flicker
[6], written in co-authorship with Dmitrieva E.N. and Topchy V.A. In this publication, the authors point out a discrepancy in the current standards between the requirements for the accuracy of the measurement of flicker doses and the range of oscillations, suggest ways to improve the verification of the flickermeter and the standardization of errors.
Also, studies of gas-discharge lamps and the possibility of their supplying high-frequency voltage to reduce pulsations of the light flux were carried out. The description of the obtained results can be found in the article called Efficiency of application of high frequency voltage for supply of gas-discharge lamps
[7], written by Kuren E.G. and Harutyunyan A.G.
In this paper, the method of determining the dependence of labor productivity on the coefficient of light pulsation was improved (F.M. Chernilovskaya).
As is known, the universal index is the dose of pulsation [8], [9], which, like the dose of the flicker, assesses the additional fatigue of a person, but in a different frequency range.
The studies were carried out on the basis of an appropriate pulsation model, based on a weighing filter 1 (Figure 2), which simulates the reaction of a person's sight to the change in instantaneous values of illumination e(t). Human's fatigue is modeled by a quadrator 2 and a link 3 of ten-minute averaging.

Figure 4 – Model of the pulsation of the light flux
Initially, the experimental values were the values of labor productivity for single-, two- and three-phase switching of lamps, and for 100% the productivity was accepted for single-phase switching on. During the experiments, the pulsation coefficients were recorded at frequencies of 100, 200 and 300 Hz, i.e. in fact, the dose ratios of pulsation. The following values of coefficients and productivity were getting: 73 и 100; 40 and 115,8; 13 and 138,6 %.
By the method of least squares, the experimental data were approximated by the authors:
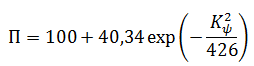
with an error of less than 0,9%.
The design capacity refers to the case of operation with unchanged illumination. It is natural to take this productivity for 100%. With a zero dose factor, the formula gives the value 140, 34%. Introducing the normalization factor 100 / 140.34, we obtain the required dependence, which is valid for pulsations of any form:

This dependence was illustrated by the example of fluorescent lamps. Also, several variants of power supply schemes for lamps were examined:
- Scheme without frequency converter.
- Scheme with individual frequency converter.
- Scheme with a group frequency converter at an increased frequency.
- Scheme with common rectifier and individual inverters.
The technical and economic comparison showed that the variant with an intermediate DC network gives the greatest economic effect: in comparison with the scheme with a group frequency converter it is 13, 64% more effective. The use of DC networks is possible not only for lighting supply, but also for computers and control systems. In this case, the efficiency will be even higher.
As a result of the work done, the authors came to the following conclusions:
- 1. ПThe use of increased frequency for powering gas discharge lamps is effective not only for industries with intense visual work, but also for outdoor lighting systems.
- 2. To supply lamps according to the scheme with a group frequency converter, a frequency of 400 Hz is recommended. Frequency for feeding lamps with individual frequency converters is not limited.
- 3. The application of lighting systems with a common rectifier, as well as with a DC network and individual inverters is promising.
Conclusion
In this paper, information on the pulsation of various types of lamps will be collected and evaluated, an electronic database on pulsations of their light flux is created, and a dynamic model of electric light sources will be proposed. It will allow estimating pulsations taking into account the shape of the graph of the process of changing the illumination, and not only its minimum and maximum ordinates.
When writing this essay, the master's work is still under development. Final concluding: June 2018. The full text of the work and materials on the topic can be obtained from the author or his supervisor after the specified date.
References
- Рончи Л. Р. – Зрительные и биологические воздействия света в новом тысячелетии: предложения для образования. //Светотехника. – 2005. – №6, С. 24-27.
- Г.К. Брейнард, И. Провенсио. Восприятие света как стимула незрительных реакций человека. //Светотехника. – 2008. - №1, С.6-12.
- В. Ван Боммель. Результаты последних исследований и их значение для светотехнической практики. //Светотехника. – 2005. - №4. С.4-6.
- Осветительные установки. – Л.: Энергоиздат. Ленингр. отд-ние, 1981. – 288 с., ил.
- Kaija Polak, Leopold Schmetterer, Charles E. Riva. Influence of Flicker Frequency on Flicker-Induced Changes of Retinal Vessel Diameter. – Investigative ophthalmology & visual science, August 2002.
- Дмитриева Е.Н., Куренный Э.Г., Топчий В.А. Погрешности расчета доз фликера напряжения и проверка функционирования фликерметра. // Электричество. - 2013.
- Куренный Э.Г., Арутюнян А.Г. Эффективность применения напряжения повышенной частоты для питания газорязрядных ламп. // Наукові праці ДонНТУ – Електротехніка і енергетика – Випуск 67 – 2003.
- Куренный Э.Г., Шидловский А.К., Арутюнян А.Г. Динамические модели электромашнитной совместимости электрических источников света. – Техническая электродинамика, 1985, №2. – С. 12-16.
- Куренный Э.Г., Дмитриева Е.Н., Куренный Д., Цыганкова Н.В. Совершенствование фликер-модели. – Электричество, 2003, №2. – С. 17-23.