Abstract
Content
- Introduction
- 1. Theme urgency
- 2. Goal and tasks
- 3. Distortion sine wave
- 4. Model replacement capacitor
- 5. Rationing non-sinusoidal voltage
- 6. Reducing non–sinusoidal voltage
- Conclusion
- References
Introduction
Electromagnetic Compatibility (EMC) – the ability of an electrical receiver (receiver) to function normally in electromagnetic environment and not interfere with this medium, which is unacceptable for other receivers Applied to problems of power supply under the electromagnetic environment refers to the power supply network to which the receiver group is connected.
The problem of EMC is in a certain sense similar to the problem of environmental protection: increasing capacity electrical receivers and the intensification of their modes of operation lead to a distortion of the parameters of electrical energy, which, in turn, adversely affects the modes of operation of other network power consumers. Security EMC is costly, with high demands on accuracy and the validity of EMC assessment methods in power supply networks.
Ensuring electromagnetic compatibility is one of the main requirements for power supply systems. The overestimation of EMC estimates leads to an unjustified increase in investment, and an understatement leads to damage from additional energy losses, reducing the service life of electrical equipment, deterioration of product quality. Due to this high Requirements are made for the validity and accuracy of EMC assessment methods both at the design stage and in operation power supply systems.
In this paper, EMC will be considered only from the standpoint of non–sinusoidal voltage. Non–sinusoidal regimens adversely affect the operation of power electrical equipment, relay protection systems, automation, remote control and communications. Resulting from impact of higher harmonics economic losses are mainly due to the deterioration of energy performance, reduced reliability functioning of electrical networks and reducing the life of electrical equipment. And since the number of non–linear loads is constantly growing, then the problem of the influence of non–sinusoidality on electrical equipment becomes more acute with each passing year.
1. Theme urgency
Condenser units (CU) are one of the most sensitive to non–sinusoidal electrical apparatus, therefore, the study of the influence of higher harmonics is a very topical issue.
2. Goal and tasks
The purpose of this work is to analyze the possible options for studying the effect of non–radial voltage on capacitor installations; consideration of the problems of electromagnetic compatibility, as well as the analysis of current methods for studying the effect of non–sinusoidal voltage (higher harmonics) on capacitor banks.
3. Distortion sine wave
Electromagnetic interference propagating through the elements of the electrical network, called conductive. Since the work deals only with non–sinusoidal voltage, the term interference will be attributed to the distortion of the sinusoid.
Non–sinusoidal voltage is the most common conducted EMC disturbance. In most publications, this interference is estimated for private cases of periodic distortions of the voltage curve. However, in existing networks, interference is a random process, which requires the development of common methods of analysis.
It is necessary to develop a universality of approach, when there are no restrictions on the type of interference random, periodic or constant processes.
4. Model replacement capacitor
Consider the simplest capacitor install. The simplest model of CU is the ideal C capacity. It is usually used in cases where the distortion of the sinusoid occur in relatively small frequency range.
For example, in[4] the number of harmonics taken into account is 40, which corresponds to a maximum frequency of 2000 Hz. In this range, such a simple model is quite acceptable.
The design uses the calculated noise graphs with rectangular dips in the sine wave. In this case, the ideal capacity cannot be used, since the derivative vertical jump equals infinity. In this connection, dynamic models of capacitors are used.
Since it is rather difficult to obtain an exact mathematical description of such a system, they often use simplified dynamic models based on an equivalent circuit. a capacitor corresponding to the physical nature of the processes occurring in it. In accordance with [ 5 ], the capacitor replacement circuit has the form shown in fig. 1.
Here: r is the active resistance of current–carrying parts; L is the equivalent inductance of a capacitor, approximately equal to the sum of the inductances of the terminals of the connecting busbars and self–inductance section; R, C – respectively active and capacitive resistance of the dielectric.
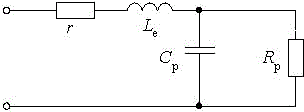
5. Rationing non–sinusoidal voltage
Non–sinusoidal voltage is a normalized indicator in accordance with GOST 32144–2013 (quality standards of electrical energy in general–purpose power supply systems).
Characterized by the following indicators:
– harmonic voltage component KU(n);
– total harmonic voltage componentKU;
According to GOST 32144–2013, harmonic voltage components are usually caused by non–linear loads of users of electrical networks connected to electrical networks of different voltage. Harmonic currents flowing in electrical networks create voltage drops on the impedances of electrical networks. Harmonic currents, impedances of electrical networks and, consequently, the voltage of the harmonic components at the points of transmission of electrical energy change over time. For example, such non–linear loads can be:
- Valve converters;
- Thyristor–controlled power equipment;
- Arc and induction electric furnaces;
- Fluorescent lamps;
- Installation of arc and resistance welding;
- Frequency converters;
- Household appliances (computers, televisions, etc.).
In batteries, current harmonic capacitors also lead to additional energy losses. As a consequence, there is an additional heating of the condenser, which can cause to the exit of the last system. Damage to the capacitor is also possible if harmonic resonances occur in the network.
The distortion of the shape of the voltage curve activates the occurrence and flow of ionization processes in the insulation of the EC, while developing local defects in the insulation, which leads to to increase dielectric loss and reduce service life.
In the presence of higher harmonics (VG) in the voltage curve, the aging process of the capacitor dielectric also proceeds more intensively than in the case when the capacitors operate at sinusoidal voltage. This is explained by the fact that physicochemical processes in dielectrics, which cause their aging, are significantly accelerated at high frequencies fields. Similarly affects the additional heat caused by the flow of HG current.
Capacitor batteries can operate for a long time when overloaded with VG currents by no more than 30%; allowable voltage increase is 10%. However, under these conditions, their duration service is shrinking. In SES industrial enterprises, as a rule, BK may be in the mode close to the resonance of currents at the frequency of any of the SH; due to overloads they fail.
Many countries in Western Europe use capacitors manufactured by Merlin–Gerin. Polypropylene is used as a dielectric. Mode of operation of these capacitors at voltage 1,1×Uном and current 1,3×Iном as in the presence of non–sinusoidal voltage. Therefore, special filter capacitors are not produced. Operating experience BK within the guaranteed time of reliable work (resource) confirmed their high performance; so detailed studies of the aging of a dielectric capacitor, Filter compensating devices used in circuits are not always implemented.
6.Reducing non–sinusoidal voltage
Reducing the non–sinusoidal voltage is provided either by a rational construction of the enterprise SES, at which the parameters characterizing the non–sinusoidal voltage, will be within acceptable limits, or the use of special switching circuits of nonlinear loads, as well as corrective devices. Non–sinusoidal voltage reduction methods can be divided into three groups:
a) circuit solutions: the allocation of non–linear loads on a separate system of tires, the dispersion of loads on various nodes of the SES with the connection of electric motors parallel to them, grouping the converters according to the phase multiplication scheme, connecting the load to the system with greater power, a) circuit solutions: separation of nonlinear loads on a separate system tires, the distribution of loads on various nodes of SES with the connection of electric motors parallel to them, the grouping of converters according to the phase multiplication scheme, the connection of the load to a system with more power;
b) the use of filtering devices, the inclusion in parallel of the load of narrow–band resonant filters, the inclusion of filter–compensating devices (PKU), the use of filter–symmetrizing devices (FSU), the use of high–speed static sources of reactive power (SRP) containing PKU;
c) the use of special equipment, characterized by a low level of generation of higher harmonics, the use of "unsaturated" transformers, the use of multiphase converters with improved energy performance.
In practice, as a rule, various methods are combined.
The development of the element base of power electronics and new methods of high–frequency modulation led to the creation in the 70s of a new class of devices that improve the quality of electric power – active filters (AF). Immediately, a classification of active filters into sequential and parallel, as well as current and voltage sources arose, resulting in four basic circuits.
Each of the four structures (Fig. 2. б) defines a filter scheme at the operating frequency: keys in the converter and the type of keys themselves (bidirectional or unidirectional key). As storage energy in the converter, which serves as a current source (Fig. 2.a, в), inductance is used, and in the converter, which serves as a voltage source (Fig. 2. б, в), capacitance is used.
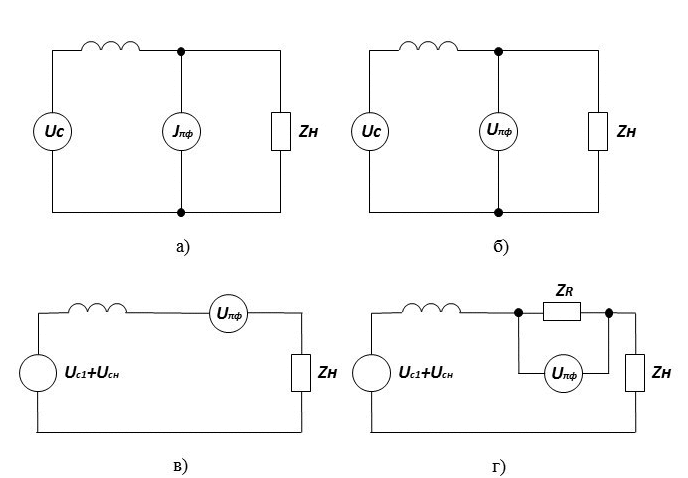
Such a filter, which, in addition to filtering the harmonics, will generate reactive power, and compensate for power losses in the network and voltage, is called filter compensating (FC).
From the above it follows that the devices of the type FC and FSU act simultaneously on several indicators of the quality of electrical energy (non–sinusoidality, asymmetry, voltage deviation). Such devices for Improvements in the quality of electrical energy are called multifunctional optimizing devices (MOD).
The expediency in the development of such devices arose due to the fact that sharply alternating loads such as arc steel–smelting furnaces cause a simultaneous voltage distortion in a number of indicators. Application MOD allows you to comprehensively solve the problem of ensuring the quality of electricity, i.e. simultaneously on several indicators.
The category of such devices includes high–speed static sources of reactive power (SRP).
According to the principle of controlling reactive power, the SRP can be divided into two groups: high–speed static sources of reactive power, direct compensation, high–speed static sources of reactive indirect compensation capacity. The structures of the SRP are presented respectively in Figure 3, a, b. Such devices, possessing high speed, allow to reduce voltage fluctuations. Phase Regulation and Availability filters provide balancing and reduction of higher harmonics.
In fig. 3, a direct compensation circuit is presented, where a controllable
source of reactive power is a capacitor bank switched with thyristors. The battery has several sections and allows
discretely change the generated reactive power. In fig. 3, b, the power of the SRP is changed by means of reactor control. With this method of control, the reactor consumes an excess of reactive power generated by the filters.
Therefore, the method is called indirect compensation.
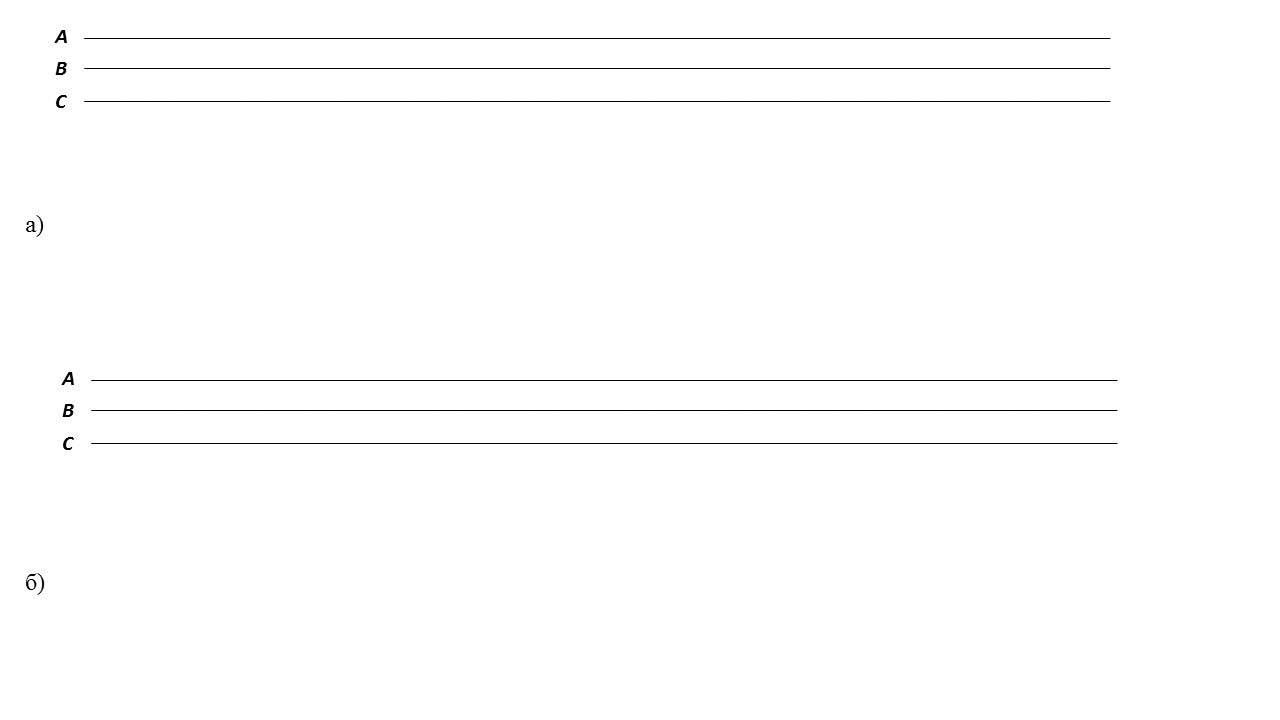
Indirect compensation has two major drawbacks: the absorption of excess power causes additional losses, and the change in reactor power using the angle of control of the valves leads to additional generation of higher harmonics.
Conclusions
- Distortions in the shape of the stress curve have a significant negative effect on the capacitor bank.
- Existing methods for assessing these impacts are not universal.
- A universal model is required with the ability to set CU parameters and the type of interference.
At the time of writing this essay, the master's work is not yet completed. Estimated completion date of master's work: June 2019. The full text of the work and materials on the topic can be obtained from the author or his head.
after the specified date.
References
- Введение в статическую динамику систем электроснабжения/ Шидловский .К., Куренный Э.nbsp;Г. – Киев: Наукова думка, 1984. – 273nbsp;с.
- Электромагнитная совместимость электроприемников промышленных предприятий/ Под ред. А.К. Шидловского. – Киев: Наукова думка, 1992. – 236nbsp;с.
- Электромагнитная совместимость. Несимметрия и несинусоидальность напряжения/ Кузнецов .Г., Куренный Э.Г., Лютый А.П. – Донецк: Норд–Пресс, 2005 – 250nbsp;с.
- ГОСТ 13109–97. Межгосударственный стандарт. Электрическая энергия. Совместимость технических средств электромагнитная. Нормы качества электрической энергии в системах электроснабжения общего назначения. – Введ. в Украине с 01.01.2000.
- Комлев В.П., Малафеев С.И. Динамическая модель силового конденсатора и ее применение для расчета потерь при искажениях напряжения. – Владимир, 1982. – 12nbsp;с. – Деп. в Информэнерго 29.11.82, №1196эн – Д82.
- Коломытцев А.Д. Динамические показатели электромагнитной совместимости электрооборудования с системами электроснабжения промышленных предприятий по несимметрии и несинусоидальности напряжения. – Автореферат на соиск. уч. степени канд. техн. наук. – Донецк: ДПИ, 1993. – 24 с.
- Малафеев С.И. О динамических и энергетических характеристиках силовых конденсаторов // Оптимизация систем питания и электрооборудования электротехнологических установок: (Сб. научн. трудов). – Киев: Ин–т электродинамики АН УССР, 1989. – с. 110–116.
- Конденсаторы переменного тока в тиристорных преобразователях/Ермуратский В.В., Ермуратский П.В. – М.: Энергия, 1979. – 224 с.
- Уэйкерли Д. Проектирование цифровых устройств / Д. Уэйкерли. – М.: Постмаркет, 2002. – Том 2. – 528 с.
- Breedingnbsp;K. Digital design fundamentals / K.nbsp;Breeding. – Prentice Hall, 1992. – 446nbsp;pp.Высшие гармоники в сетях промпредприятий/Жежеленкоnbsp;И.nbsp;В. – М.: Энергоатомиздат, 1984. – 160 с.