Abstract
Table of contents
- Introduction
- 1. Relevance
- 2. Goal and tasks of the research
- 3. Coal structure research review
- 3.1 Change of coal structure with metamorphism
- 3.2 The functional composition of coals and its change with metamorphism
- 3.3 Change in the structure of coal during the heat treatment
- 4. DRIFT-Spectroscopy Method
- Results and its discussion
- Conclusion
- References
Introduction
Coal is a multicomponent rock formed from the products of biochemical and physicochemical conversion of the original plant material. Changes in the organic matter of SFF under various geological conditions of their formation contribute to the existence in nature of numerous types, classes, and varieties of fossil coal, the composition and properties of which vary over a wide range. In this regard, the diversity of SFF found in nature necessitates their systematization, in which they are classified according to the most general and characteristic features. One of the tasks of coal chemistry is to create a comprehensive classification. The requirements for a modern classification of fuels are very diverse.It should be based on more characteristic features of the fuel, which would take into account the functional structure and structure of the TGI.
1. Relevance
The study of the structure of coals and their pyrolysis products is important for theoretical and practical coal chemistry, and is also directly related to the development of new international classifications of solid fossil fuels. The existing coal classifications, including the International System of Codification of Coals of medium and high ranks, are based on the determination of such characteristics as elemental and technical analysis, petrographic composition, calorific value, vitrinite reflectance, sintering ability, coking ability, etc. These marks are clearly not enough to decision the problem of rational non-fuel use of fuels. In addition to energy and coke and chemical uses, fossil coals can be widely used to produce valuable chemical products.
There are three critical characteristics that affect the properties of coal. This is the stage of metamorphism, the genetic type of recovery and petrographic composition. These characteristics are closely related to the structure of coal.
Currently, it is of great interest to study the functional and structural groups that make up coals by modern methods. Of great importance for modern coal chemistry are the processes that occur with them during metamorphism and thermal destruction. The development of principles for constructing a classification of coal on the basis of fundamental ideas about the structure of matter is very relevant. Attempts to find new coal quality indicators are being undertaken by scientists.
The available chemical and technological characteristics of coal quality need improvement with the use of modern research methods.
2. Goal and tasks of the research
The goal of the work is to assess the influence of the degree of metamorphism, the genetic type of reduction on the structure of coals and their pyrolysis products and determine the parameters that best reflect these characteristics.
Research object: coals of the Donetsk basin of different stages of metamorphism and genetic types in terms of recovery.
Research subject: determination of the parameters that best reflect the change in the structure of OMC during metamorphism and heat treatment using the DRIFT spectroscopy method.
As part of the master's work, it is planned to obtain relevant scientific results in the following direction:
- The influence of the degree of metamorphism on structural changes in OMC
- The influence of the genetic type of recovery on structural changes in OMC
- The influence of heat treatment on structural changes in OMC
- Search for classification characteristics that take into account the structural and functional composition of SFF
3. Coal structure research review
3.1 Change of coal structure with metamorphism
Coal metamorphism is the result of the conversion of organic residues under the influence of a combination of geological factors. Such factors, as a rule, include temperature, pressure, microbiological characteristics of the environment, air access, humidity, etc.
The transformation of dead plants into coal occurs as a result of a continuous process in which it is customary to distinguish two main phases:
- Humification is the transformation of dead plants into peat;
- Coalification is the conversion of peat sequentially into brown, hard coal and anthracite.
Carbonization is divided, in turn, into two parts:
- The diagenesis of coal, during which peat turns into brown coal under the influence of mainly biochemical transformations due to the vital activity of microorganisms;
- Metamorphism, during which brown coal under the influence of physical factors – elevated temperature and rock pressure – turns into coal and anthracite.
The main direction of the process in metamorphism is an increase in carbon content or carbonization. With an increase in the stage of metamorphism, the content of oxygen and hydrogen also decreases, and the moisture content decreases. The ratio of hydrogen to carbon, the yield of volatile substances is reduced. The reflection index of vitrinite increases.
However, there are marks that do not change monotonously. These marks include sintering, calorific value, solubility in pyridine. The change in calorific value has a maximum at the middle stage of metamorphism. This is due to a change in elemental composition. Low-metamorphosed coals, which contain a lot of oxygen, have the lowest value. Further, it increases as oxygen decreases and carbon increases, and in highly metamorphosed coals it decreases due to a decrease in the hydrogen content, but remains higher than in slightly metamorphosed coals.
The change in elemental composition in the process of metamorphism is due to the release of volatile substances that contain a significant amount of carbon dioxide, water vapor, methane.
Low-metamorphosed coals with a carbon content of less than 80–85% are more characterized by a colloidal rather than crystalline character. However, the colloidal structure of coals cannot explain a number of properties of highly metamorphosed coals. In the process of coalification, due to the reactions of dehydrogenation, dehydrocyclization and condensation, the proportion of aromatic carbon increases, the number of aromatic rings increases. At the same time, the supramolecular structure also changes, it is gradually being ordered: the atoms of the polymer skeleton having excess positive and negative charges interact with each other, forming donor-acceptor bonds that mutually orient the ring fragments and increase the rigidity of the skeleton. So the diameter of the lamellas increases especially rapidly when the carbon content becomes more than 90%. At the same time, the bridges are shortened and become stronger, and the functional groups are eliminated, only the ever decreasing number of the most stable phenolic groups and methyl substituents remains. With coal metamorphism, the crystal lattice is densified. Therefore, during internal crystallization, the substances that make up the coal from the initial metastable state, characterized by an inhomogeneous non-crystalline structure, go into the final stable state – the state of graphite having an ultramicrocrystalline structure.
3.2 The functional composition of coals and its change with metamorphism
Functional groups in coals are very diverse. They are represented by carboxyl, carbonyl (including quinoid), hydroxyl (alcoholic, phenolic), ether, bridging structures containing oxygen and sulfur, as well as alkyl substituents, –SH, –NH, –NH2 groups.
Data on changes in structural groups in coal showcases of different stages of metamorphism make it possible to distinguish three stages of coal structure transformation. At the first stage, coals contain many carbonyl and hydroxyl groups linked by hydrogen bonds of various strengths, framework structures and aliphatic bonds, and the amount of unsaturated С–Н bonds in the conjugation system is insignificant. The carbon skeleton includes relatively small units with a low degree of conjugation and with a high number of oxygen-containing groups. They are connected by hydrogen bonds, which lengthen fragments of frame structures. With an increase in the degree of metamorphism, a rapid decrease of number in aliphatic and oxygen-containing groups occurs, and the number and strength of hydrogen bonds decreases. The process of improving the structure occurs due to the removal of unstable oxygen-containing groups and aliphatic hydrogen, as well as the disproportionation of hydrogen, as a result of which CH3– groups and unsaturated CH– bonds are formed.
At the second stage, the amount of carbonyl groups, framework structures decreases to a minimum in coals, the number of hydroxyl groups, aliphatic hydrogen decreases, but the number of methyl groups reaches a maximum, and the number of С–Н bonds in the polyconjugation system increases. The main process at this stage is the growth of the poly-conjugation system. The intermolecular interaction due to hydrogen bonds is also significantly weakened.
At the third stage, the transformation of the structure is due to the improvement and growth of the poly-conjugation system. The number of unsaturated С–Н bonds, which are formed due to a decrease in aliphatic hydrogen, is increasing. The content of carbonyl and C=C groups increases [1].
3.3 Change in the structure of coal during the heat treatment
When heated without air, solid fuels undergo complex changes. Such heating of coal in an inert atmosphere is called pyrolysis. Thermal destruction of solid fuels is a multi-stage process in which decomposition, hydrogenation, dehydrogenation, and condensation occur in parallel and sequentially. Therefore, both complex multicore systems and simpler liquid and gaseous products are formed [2].
Some coals of the middle stage of metamorphism form a liquid phase upon heating, turning into a plastic state in a narrow temperature range. In this case, a relatively uniform mass is formed, that is, sintering occurs. Currently, it is generally accepted that the formation of a liquid phase is necessary for sintering coal. At every moment, decomposing coal coexists in the plastic mass of coal, the liquid phase undergoes changes, and a new solid phase forms. The quality of the obtained coke depends on the properties of the plastic mass and the dynamics of gas evolution during the thermal transformation of coal. The ratio of the rates of decomposition and the formation of new products at all stages of the existence of the plastic state determines the amount of the liquid phase and the fluidity of the plastic mass, which affect the caking ability and quality of coke. The curing of plastic mass – the nucleation and development of a new solid phase – occurs both on existing solid particles as a result of heteropolycondensation of the sorbed liquid phase and directly in the mass of the liquid phase during the interaction of its components. With a decrease in the viscosity of the plastic mass, the degree of ordering of the structure and the mutual orientation in space of flavored macromolecules and their blocks increase. The nuclei of a new solid phase are formed from them. At a sufficiently high concentration of nuclei, bonds arise between them, and the plastic mass is structured. In this case, the viscosity increases rapidly, and a crosslinked solid structure is formed.
4. DRIFT-Spectroscopy Method
Infrared spectroscopy has long been used as an informative method for studying the molecular structure of coal [. Modern IR spectroscopy is an express method for establishing the structural features of organic compounds. Infrared radiation is called radiation with wavelengths from 0.5 to 1000 microns. In the IR range, transitions between vibrational and rotational energy levels of molecules are manifested. Chemical bonds in molecules undergo vibrational movements. The vibrational energy of the molecules is quantized, i.e. the absorbed energy does not change continuously, but stepwise. As a result, the vibrational (infrared) spectrum of the molecule is a series of peaks (absorption bands) corresponding to different vibrational energy transitions. Most vibrational transitions in the molecules of organic compounds occur in the wavelength range λ from 2.5 to 25 ?m. In units of wave numbers ν = 1/λ (cm-1), the reciprocal of the wavelengths, this interval is 4000–400 cm-1. It is in this wave number range that the IR spectra of organic and natural compounds are recorded.
There are many different instruments for IR spectroscopy. Some of them use Fourier transforms. The Fourier transform is a complex computational procedure, however, the intensive development of computer technology has led to the creation of small-sized high-speed computers built into the spectrometer, which make it possible to obtain the spectrum and process it in a short time.
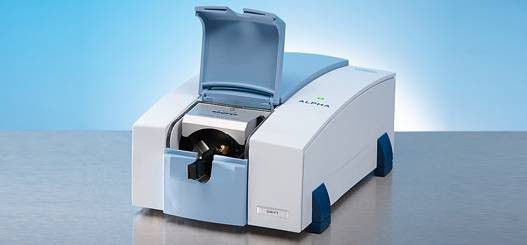
Picture 1 – DRIFT-spectrometerр
Fourier spectroscopy has a number of significant advantages. The two main advantages of interferometers over conventional spectrometers are as follows. Firstly, this is a gain in energy due to the fact that during scanning at every instant of time the receiver receives radiation from the entire studied spectral range of wavelengths, and not its narrow section, which is determined in the monochromator of a conventional device by a dispersing system and slots. In other words, in the interferometer, during the entire time of scanning, information is obtained simultaneously about the entire studied spectral range, and in a conventional spectrometer at different times, information is obtained only about narrow spectral bands of the studied range. This advantage of interferometers is especially important in the long-wavelength region, where the radiation intensity of the source is small and the signal-to-noise ratio is a limiting factor. Secondly, a big gain makes it possible to increase the resolution of the interferometer without reducing the flux of radiant energy. The resolution of the Fourier spectrometer is proportional to the maximum difference in the path of the beams, and to increase, for example, the resolution of the spectrum by a factor of two, you just need to double the length of the mirror travel, and, accordingly, the recording time.
Infrared spectroscopy, due to its ideal wavelength range, is one of the most universal methods for studying the structure of chemical compounds. Infrared radiation with a frequency of less than 100 cm-1 is absorbed by an organic molecule and converted into energy of its rotation, and radiation in the range of about 10,000–100 cm-1 is converted into the energy of vibrational movements of atoms in the molecule. Investigations in the field of IR spectroscopy of fuels made it possible to establish the similarity of the structure of coals and their products [9].
The improvement of instrumental methods of analysis recently led to the emergence of highly sensitive infrared spectroscopy with Fourier transform (FT-IR). The possibility of obtaining high-quality diffuse reflectance spectra of coal (DRIFT) allows charcoal to be characterized much faster than conventional methods [8, 9].
Fourier transform infrared spectroscopy is an infrared spectroscopy method that uses an interferometer rather than a conventional system of diffraction gratings and narrow slits. The initial result of the scan is an interferogram, which is connected with the IR spectrum by a mathematical operation known as the Fourier transform. The big advantage of the FT-IR method is the ability to use a computer for digital data accumulation and processing, which allows performing the operations of expansion and contraction of spectra, their comparison or synthesis, factor and correlation analysis, subtraction of the spectrum of solvent or mineral impurities, showing and printing of curves, programmed control experiment, baseline correction. The baseline is drawn through the points of local minima on the absorption curve. The baseline method allows you to partially eliminate the consequences of deviations from the Lambert-Behr law due to the heterogeneity of the samples, the presence of larger particles compared to the wavelength, etc.
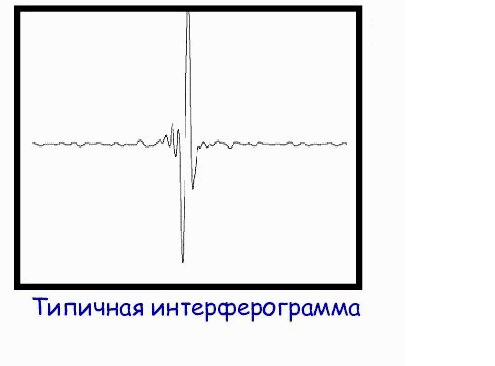
Picture 2 – Converting Interferograms to Spectrum (animation: 10 frames, 5 cycles of repetition, 51 kilobytes)
5. Results and its discussion
The paper shows the difference in the structural-group composition of coals of different stages of metamorphism and different genetic types in terms of reduction and their pyrolysis products.
It has been established that in the temperature range of semicoking, the bridge bonds are destroyed, aliphatic hydrogen is removed as a result of dealkylation reactions, as well as the accumulation of the most stable aromatic structures and C = O groups. In this case, aliphatic and oxygen-containing groups are partially preserved in the coke of gas coals. Sulfur coal cokes are richer in oxygen-containing groups, contain a greater number of short aliphatic chains and are characterized by significantly greater intermolecular interaction strengths compared to low-sulfur coal cokes. Consequently, said cokes will have higher mechanical strength.
The data presented show the wide possibilities for assessing the quality of solid pyrolysis products by DRIFT spectroscopy.
It has been experimentally shown that the main parameter characterizing metamorphism is the relative content of phenolic groups, and the parameter characterizing sintering ability – the ratio of the content of oxygen-containing groups to aliphatic chains.
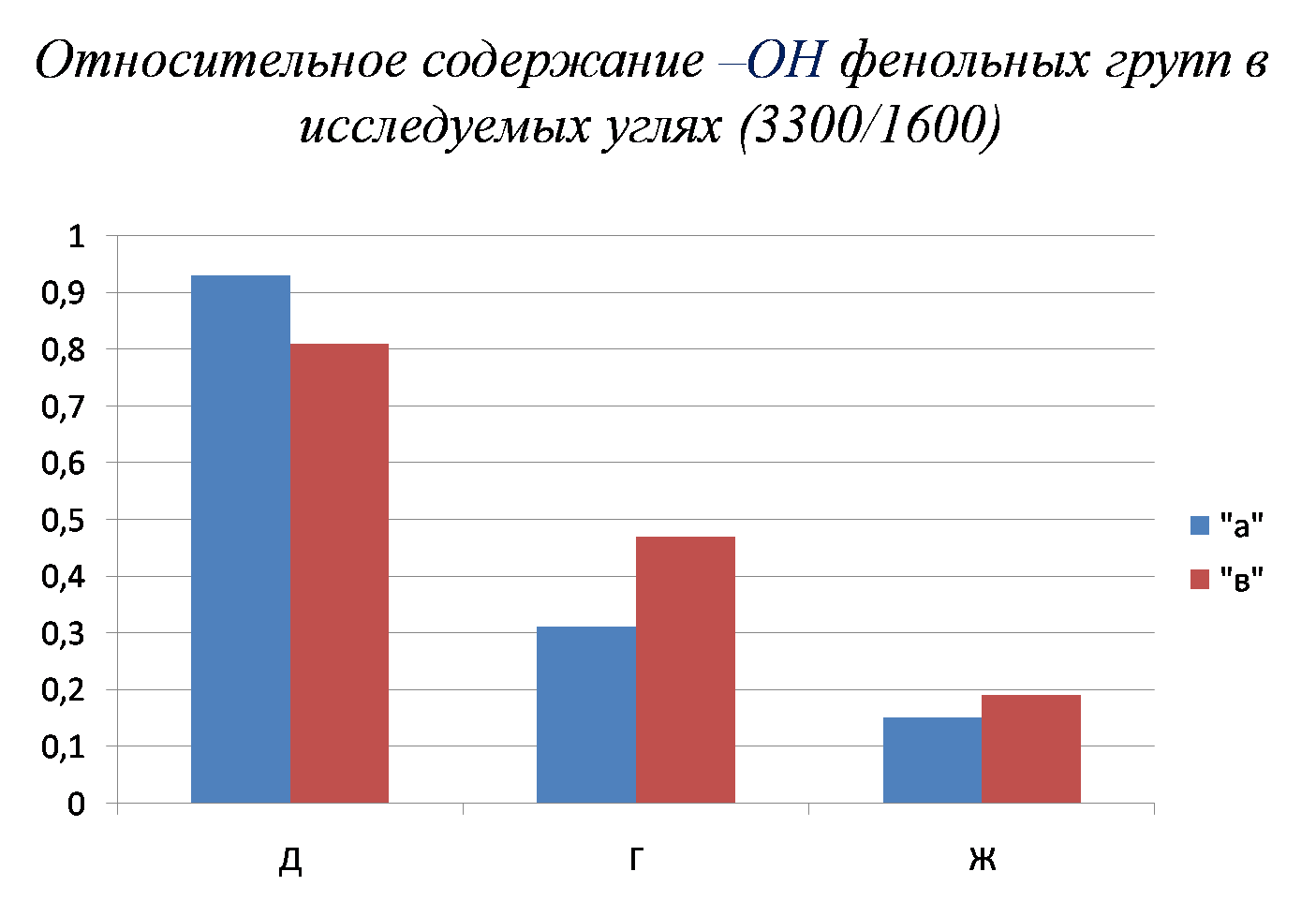
Picture 3 – The relative amount of phenolic groups (а – slightly reduced coals; в – reduced coals)
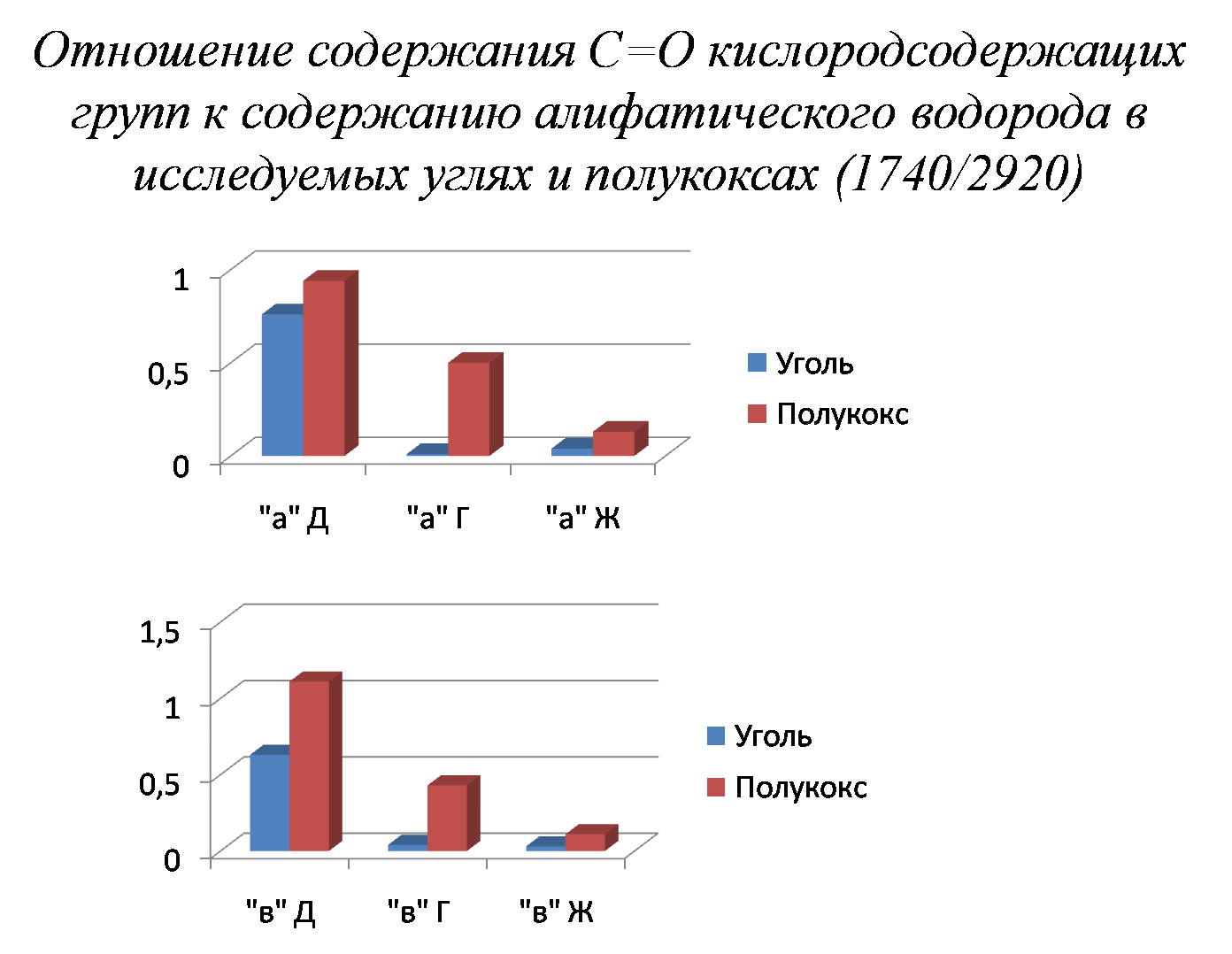
Picture 4 – The ratio of the amount of oxygen-containing groups to aliphatic chains
Conclusion
The paper proposes new structural parameters for assessing the quality of coal and their subsequent rational use. These parameters are determined by rapid DRIFT spectroscopy.
When writing this essay, the master's work is not yet completed. Final completion: June 2020.
References
- Русьянова Н. Д. Углехимия, – М.: Наука, 2003 г. &ndsah;317 с.
- Русчев Д. Д. Химия твердого топлива, – Л.: Химия, 1976г.–254с.
- Гюльмалиев А. М., Головин Г. С., Гладун Т. Г.Теоретические основы химии угля, –М.:Издательство московского государственного горного университета, 2003г. –558с.
- По ред. д. х. н. проф. Липовича В. Г. Химия и переработка угля, –М.: Химия, 1988г.з–337с.
- Гофтман М. В. Прикладная химия твердого топлива, –М.:Государственное научно-техническое издательство литературы по черной и цветной металлургии, 1963г. –596с.
- Николаева В. И., Буваков К. В., Табакаев Р. Б. Методы исследования свойств твердых топлив. –Томск.:Издательство Томского политехнического университета, 2013г. –92с.
- Fanning P. E., Vannice A. A. Drifts study of the formation of surface groups on carbon by oxidation// Carbon. – 1993. – V.31, №6. – Р.721–730.
- Fuller M. P., Hamadeh I. M., Griffiths P. R. and Lowenhaupt D. E. Diffuse reflectance infrared spectrometry of powdered coals // Fuel. – 1982. – V.61, № 6. – P.529–536.
- Методы определения структуры угля /Вестник Кузбасского государственного технического университета. 2017. № 4, с.147–154 [Text]
- M. J. Iglesias, M. J. Cuesta, F. Laggoun-Defarge, I. Suarez-Ruiz. The influence of impregnation by hydrocarbons on coal structure during its thermal evolution, J. Anal. Appl. Pyrolysis 58–59 (2001) 841.
- M. J. Iglesias, J. C. del Rio, F. Laggoun-Defarge, M. J. Cuesta, I. Suarez-Ruiz. Control of the chemical structure of perhydrous coals; FTIR and Py-GC/MS investigation, J. Anal. Appl. Pyrolysis 62 (2002)
- Москаленко, Т. В. Теоретические методы расчета структурных параметров органической массы углей (обзор). / Т. В. Москалеко, О. С. Данилов, В. А. Михеев, А. М. Леонов // Горн. информ.-аналит.бюл. – 2009. – вып. 10. – С. 417–427.