Take a moment to look around you. Unless you are outside, you should see walls and ceilings decked out in technological artifacts: lighting fixtures, jacks (telephone and data), power outlets, and so forth. They are so common they've become a part of the landscape. Now an invisible artifact is joining this landscape. It provides high-speed, low-cost, low-power, wireless access to the Internet and other networks over a short range. It is Wi-Fi, which is a marketing term that has grown to encompass technology built upon the three latest 802.11 Physical Layer specifications: 802.11a, 802.11b and 802.11g.
In its base set of applications, Wi-Fi provides wireless connectivity via portable devices, including laptops, tablet PCs, handheld computers, personal digital assistants (PDAs), digital cameras, audio and video players, cell phones, headsets, and even portable devices that people can wear on their clothing. Wi-Fi also provides end-users wireless access to a host of new services through a topology referred to as a "wireless local area network" (WLAN), which usually consists of a wired Internet connection and more traditional wired voice and data connections.
Wireless LAN technologies (i.e. the 802.11 series of specifications) emphasize a high data speed and a range that makes using these technologies in a networked environment feasible. Typically, WLANs provide wireless links from portable computing devices to a wired LAN via access points (essentially a wireless hub), but there are also a growing number of stand-alone WLANs.
Now, what many people don't realize is that the wireless LAN's foundation is built upon wired technology. Let me explain.
Computers have shared information across a network via a wired connection since the 1980's. Initially, the physical path over which computers shared information was a wire, usually a coaxial cable or a Category 3 (and later Category 5 and 5e) cable. As users began to connect computers together to share information and common resources, it became essential that each computer agree on how to send that information across the networked computers. This need for different computers, built by different manufacturers, to share information eventually led to the development of a standard set of rules that each computer obeyed in order to communicate with one another.
The Institute of Electrical and Electronics Engineers (IEEE) formed the 802 Executive Committee to design specifications to standardize the physical path over which computers communicated. The result was an Ethernet-like standard published in 1985 by the 802.3 Working Group. This first publication was named "IEEE 802.3 Carrier Sense Multiple Access with Collision Detection Access Method and Physical Layer Specifications." The local area network (LAN) standard defined in that document is more commonly known as "Ethernet," although IEEE doesn't refer to 802.3 as "Ethernet," because Ethernet is a specific product trademarked by Xerox, whereas 802.3 is a set of standards. The 802.3 standard, albeit with many extensions, still governs how computers communicate today.
As the need for user mobility increased along with the cost of installing a network's cables, the business community demanded alternative methods to network its computers. The result was the development of wireless connections that use radio frequencies to transmit information.
In 1990, the IEEE 802 Executive Committee established the 802.11 Working Group to create a wireless local area network standard to govern how computers communicate over a wireless connection. The goal of that Working Group was to describe a wireless LAN that delivers services commonly found in wired networks, i.e. high-speed throughput, reliable data delivery, and continuous network connections.
The resulting 802.11 series of standards (which are in many ways extensions of the 802.3 standard) define a Medium Access Control (MAC) sublayer (of the Data Link Layer) and three Physical (PHY) Layers. The MAC sublayer is mostly made up of software-based protocols that enable devices to talk to each other and to wired local area networks. The PHY Layer defines the physical characteristics of the radio signal, i.e. the frequency, power levels, and type of modulation.
All Wi-Fi networks have an architecture that is specifically designed to support a network where all decision-making is distributed across the network's stations (i.e. the components, which may be mobile, portable, or stationary, that connects to the wireless medium). The building blocks of all Wi-Fi networks include:
1. Support of all station (computer, printers, scanners, etc.) services including authentication, de-authentication, privacy, and delivery of the data (MAC service data unit).
2. Basic Service Set (BSS), a set of stations (e.g. computing devices) that communicate with one another. When all the stations in the BSS communicate directly with each other and there is no connection to a wired network, the BSS is called an "Independent BSS" (IBSS), although it is more commonly known as an "ad hoc network" (i.e. it is typically a short-lived network with a small number of stations in direct communication range). When a BSS includes an access point (AP), the BSS is no longer independent and is called an "infrastructure BSS" or simply "BSS." In an infrastructure BSS, all computing devices (stations) communicate with the AP. The AP provides both the connection to the wired LAN (if there is one) and the local relay function within the BSS.
3. Extended Service Set (ESS), a set of Infrastructure BSSs, where the APs communicate among themselves to forward traffic from one BSS to another. The APs perform this communication via what is referred to as a Distribution System (DS). The DS is the backbone of the WLAN and may be constructed of either wired or wireless networks.
(See Fig. 7.7 for a visual explanation of each of the above-referenced Basic Service Sets.)
As explained in previous chapters, the original 802.11 standard defines the OSI's (Open System Interconnection) Data Link Layer's MAC (Medium Access Control) sublayer and PHY (Physical) Layer. And, to date, all of the ensuing amendments and supplemental standards either enhance the original MAC for QoS (Quality of Service) and security, or extends the original PHY for high-speed data transmission.
The design of 802.11's MAC interface means it is compatible with 802.3 networks. But the 802.11 MAC offers many other functions as well, including:
1. Providing a reliable delivery mechanism for user data over wireless media, which is achieved through a frame exchange protocol at the MAC level.
2. Controlling access of the shared wireless media via two different access mechanisms: the contention-based mechanism, called the distributed coordination function (DCF), and a centrally controlled access mechanism, called the point coordination function (PCF).
3. Protecting the data it delivers, which is done through a privacy service, called Wired Equivalent Privacy (WEP) that encrypts the data sent over the wireless medium.
Furthermore, Physical Layer dependent parameters (like timing intervals and backoff slots) are also modeled in the MAC.
ACCOMMODATING DIFFERENT APPLICATIONS
As applications using the 802.11 specifications broaden, e.g. HotSpots, integration into 2.5G and 3G cellular phone devices, support for streaming media, and more, a different implementation focus will be needed. All 802.11 implementations are comprised of four key components: the PHY, the MAC (both are normally delivered via a silicon chip), the Distribution Services, and the Management Services (the latter two are software components that enable 802.11 Access Points and Gateways to be created). And while all implementations of 802.11 require a MAC and PHY to operate, Management Services and Distribution Services are only required in certain applications. For instance:
The MAC and PHY components implement the 802.11 standards for MAC and PHY.
The Distribution Services provide the functionality to enable communication with a wired and wireless infrastructure via routing of frames that provide scalable, reliable and secure networks.
The Management Services provide the ability to manage a large-scale wireless infrastructure, to monitor performance, to determine the best method to tune network performance, and to modify operational parameters such as security and Quality of Service.
|
Wireless LANs can provide network flexibility and make it easy to support a roving workforce, but pitfalls abound. For instance, you must decide which flavor of 802.11 technology to select. Not long after the original 802.11 specification was ratified in 1997, the 802.11 Working Group formed two Task Groups, 802.11a and 802.11b, to work on extensions to the PHY Layer of the 802.11 specification and to solve some of the shortcomings of that original specification. The resulting 802.11a and 11b specifications also were designed to work with the original 802.11's MAC sublayer. But that's where much of the resemblance between these two standards ends.
To help in your decision-making process, we will now consider some of the options, security concerns, and the pieces of the puzzle you'll need to consider when evaluating a WLAN deployment, at least as things stand at this writing.
Once 802.11b gear hit the marketplace in 1999, network managers chose it as their preferred WLAN standard, mainly because there were no significant contenders since manufacturers were slow to produce any quantity of 802.1 la gear. But by late 2001 everything changed as vendors released 802.11a access points and radio network interface cards. This change meant that anyone considering the deployment of a WLAN had another viable wireless technology to consider. Then in 2003 the manufacturers "jumped the gun" by releasing 802.11g gear in advance of a finalized set of specifications upon which to build that gear. Of course, now that 802.11g has been finalized expect also to find Wi-Fi certified 802.11g products all over the place.
Nonetheless, 802.11b is still the technology in current favor. And while some industry experts believe that 11a might be the anointed successor to 802.11b, others believe that since the 802.11g specification has been finalized, 11a may find itself out in the cold. So the decision on which specification to use when deploying a new WLAN can get a bit complicated.
Since 802.11g is spanking new and thus products built upon that standard do not have the experience behind them that only an installed base can provide, we will consider the major points of differentiation between 11a and 11b. However, there is an extensive discussion of the new specification in Chapter 6 and a discourse on the challenges of adding 802.11g to an existing 802.11b network in Chapter 10.
It's only through the examination of the main technical differences between 802.1 la and 802.11b specifications that it is possible formulate a choice between 802.11b (and its 802.11g extension) and 802.11a. (Some may even opt for adopting a mixed environment.)
In 802.11b's favor: unlike 802.11a, 11b's products were introduced into the marketplace in 1999. Next, 802.11b's throughput is adequate for many network scenarios. Since the technology has evolved through several generations and has been used in numerous real-world situations, it has had most of the kinks worked out and its networking gear has come down to near-commodity prices. Finally, almost all HotSpot technology is based on 802.11b.
As Fig. 7.1 indicates, products with the 802.1 la designation offer higher bandwidth and more channels than 802.11b products. But before you upgrade (or before you go wireless for the first time), there are some traps to be aware of.
Figure 7.1: Technical differences between 802.11a and 802.11b.
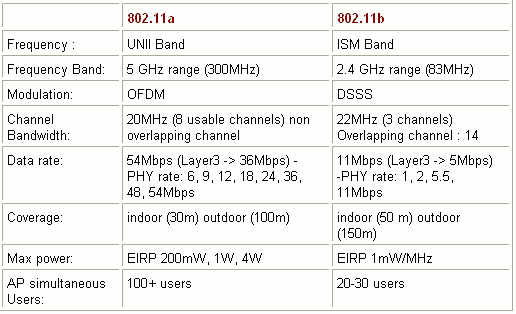
Note: This analysis uses published information, published data and the system performance parameters readily available from the FCC, the IEEE and the International Telecommunications Union (ITU). It also tries to clear up some of the confusion that has been perpetuated through the information that's been available to date, much of which, in the author's opinion, misinforms about the actual performance and network capacity of both 11b and 11a technologies.
Everyone who compares these two technologies finds major points of differentiation, but when the two are examined more closely there is no clear "winner." They each have different operational criteria.
To assess the network capacity of a wireless LAN built upon each specification, you must first understand that these two standards have different physics and operational characteristics such as:
1. The possibility of interference-although the scale may tilt a bit in favor of 802.1 la there is little difference between the two.
2. Throughput-802.11a is much higher than 802.11b's throughput, but in many instances the throughput difference isn't that important because of the applications that will be run over the WLAN.
3. Decipherable Signal Range, i.e. achievable communication range between the AP and the station, and the corresponding service coverage area-802.11b wins this contest; but in some instances, such as an area that routinely hosts a multitude of wireless users, 802.11a would be more desirable than 802.11b.
Then you must consider the different value propositions that these standards offer. They touch on many different areas including:
1. Different end-user values by segments (e.g. corporate offices have different requirements than R&D departments).
2. Usage models (e.g. a large, but busy, corporate conference room has different networking requirements than a warehouse).
3. Applications (word processing has different bandwidth requirements than videoconferencing).
4. Existing WLAN equipment (e.g. is there an existing 802.11b network?).
Now let's examine the differences between these two technologies as they pertain to the average corporate network's needs.
Interference: There are two "givens" when considering the possibility of interference in the operation of an 802.11a or b WLAN. The first concern is the unlicensed bands that 11a and 11b use, and the interference that might exist between all of the different devices that use that spectrum. The second is that given the cost of licensed spectrum, free spectrum is, and always will be, very attractive. Slivers of frequency that may not now be overcrowded will, in the near future, become so. Therefore, the claim that a technology such as 802.11a utilizes "un-crowded spectrum" is not a relevant buying consideration. Both the 2.4 GHz and the 5 GHz bands are subject to overcrowding and interference, and are used by many devices outside the WLAN arena.
The 2.4 GHz band (2.400-2.4835) is used by, not only, 802.11 and 802.11b devices, but also another WLAN technology, HomeRF. In addition, this ISM band is also used by communication systems such as Bluetooth, proprietary cordless phones, and non-communication devices, e.g. microwaves and lighting devices.
The 5 GHz band (5.15-5.25 GHz, 5.25-5.35 GHz, and 5.725-5.825 GHz), which at the moment appears less crowded than the 2.4 GHz band, hosts an ever-increasing amount of traffic. In addition to 802.11a devices, communication systems such as satellite systems (mobile and earth exploration), short range wireless systems, radio location systems, and electronic news gathering systems use the 5 GHz frequencies. The main non-communication device utilizing the 5GHz band is radar.
Throughput: An important consideration when determining which WLAN technology to use is the amount of bandwidth, data rate, or throughput the technology provides to each network user, and how well that throughput can support the applications running on the network.
Note: For our purposes, data rate is the amount of data that can be sent from one node on the wireless network to another, within a given timeframe-usually seconds, e.g. 11 Megabits per second or 11 Mbps. The difference between data rate and throughput is the measure of raw bits traveling from one node to another, in comparison to the bits representing the message content. This difference is determined by a number of factors including the latency inherent in the PHY components of the radio, the overhead and acknowledgement information that accompany every transmission, and pauses between transmissions.
To help put data rate and throughput numbers into perspective, consider this:
Figure 7.2: Speed comparisons of various connectivity technologies.
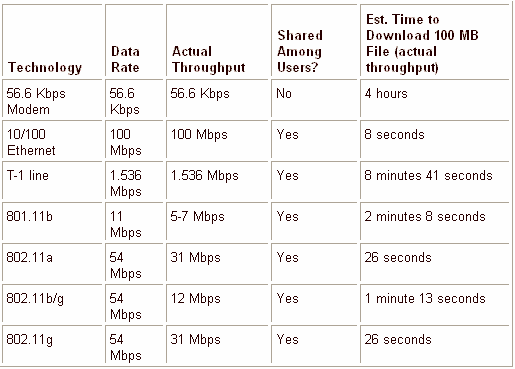
As you know, 802.11b offers a maximum data rate of 11 Mbps, which translates into approximately 5 to 7 Mbps of actual throughput, while 802.11a offers a 54 Mbps data rate, or approximately 31 Mbps of actual throughput. But, keep in mind when considering throughput rate that:
1. It is shared among all network users who use it simultaneously.
2. The throughput is managed through a CSMA/CA (Carrier Sense Multiple Access/Collision Avoidance) technique modeled on its wired equivalent (i.e. Ethernet).
3. Most network traffic (wired and wireless) is bursty, and there are typically only a few users on the network at any one time, so all WLAN networks (whether using 11a or 11b technology) offer their users generally very good connectivity speeds.
An 802.11a network can easily support several simultaneous streaming media data streams and still have enough capacity to serve other end-users with high wireless data rates. Conversely, 802.11b networks are limited in the types of applications that they can host.
Decipherable Signal Range: One of the most fundamental and significant differences between communication systems operating at 2.4 and 5 GHz is the achievable communication range between the AP and its computing devices, and the corresponding coverage area-the decipherable signal range. As you shall learn, when comparing each specification's decipherable signal range, both 802.1 la and 802.11b wireless systems are governed by the same variables.
Without delving deeply into the theories of electromagnetic wave propagation, we will look at the propagation performance (refers to the movement of the transmission signal through the airways) in the ISM and U-NII bands. Here's what you need to understand about decipherable signal range.
Because the 802.11a carrier frequency is more than twice as high as the 802.11b carrier frequency, the electromagnetic propagation through the channel should theoretically attenuate the signal twice as much (6 dB according to the so-called Friss equation). While this holds true in an "open field" situation, in an indoor or campus environment, additional parameters must be considered, such as reflection, wall penetration, moving vehicles, how fast the channel changes, and so forth. Thus, while the laws of physics dictate that the range of free-space (i.e. open field) radio communications decreases with higher frequencies, indoor propagation differs from free space because of absorption and reflections. Moreover, power transmit levels and the type of modulation used also affect range. The result is that it is very difficult to arrive at a generic formula to accurately describe such propagation.
Note: The Friss equation gives the free space power received by an antenna. This equation helps in finding out the received signal strength at the receiver side when there is an unobstructed line of sight path between the transmitter and receiver. The function is of the form
Pr = Pt*Gr*Gt*{lambda}^2/(16*{pi}*{pi}*d*d*L)
where Pr is the power of the signal at the receiver, Pt is the power of the signal at the transmitter, Gt is the transmitter antenna gain, Gr is the receiver antenna gain, d is the separation between the antenna and the receiver, {lambda} is the wavelength and L is the system loss factor which is assumed to be 1 for practical purposes.
Various models, techniques and analyses have been created to give some insight into the propagation question, including:
1. The Motley-Keenan model (J.M. Keenan, A.J. Motley, "Radio Coverage in Buildings," British Telecom Technology Journal, Vol. 8, No. 1, January 1990, 19-24).
2. The Kamerman path loss model (A. Kamerman, "Coexistence between Bluetooth and IEEE 802.11 CCK Solutions to Avoid Mutual Interference" Lucent Technologies Bell Laboratories, Jan. 1999, also available as IEEE 802.11-00/162, July 2000).
3. Long-distance models (T. Rappaport, Wireless Communications, Prentice Hall, New Jersey, 1996).
4. Multi-breakpoint models (D. Akerberg, "Properties of a TDMA Picocellular Office Communication System," IEEE Globecom, December 1988, 1343-1349).
5. Sophisticated ray-tracing techniques (K. Pahlavan, A. Levesque, Wireless Information Networks, J. Wiley & Sons, Inc., New York, 1995).
Unfortunately, some of the indoor models suggest that a carrier frequency that is twice as high will attenuate the signal by 6 dB, whereas other models reach an entirely different conclusion. While there are many reasons for such contradictory conclusions, the primary explanation is that there are a number of variables that govern the decipherable signal range of every wireless system, whether 802.11-based or not. Some considerations are:
1. RF power transmit level, the power at which the signal is transmitted.
2. Required ES/N0, the signal energy required to recover the transmitted symbol (the technical wireless term for the information contained in a message) compared to the environmental noise. (Because symbols are shorter at greater throughput levels, they require more energy in the symbol to recover it for the same error rate. This is one reason a WLAN system's throughput decreases as the distance between the AP and its computing devices grows.)
3. Environment, the physical characteristics of the radio's environmental surroundings affect the path loss.
4. Signal propagation, the physics of the radio spectrum and frequency in which the radio operates.
But the core difference between communication systems operating at 2.4 and 5 GHz is the achievable communication range between the AP and the computing device, and the corresponding service coverage area. Assuming common environments and system operating parameters, systems operating in the 2.4 GHz frequency band offer roughly double the range of those operating in the 5 GHz band, again holding power and throughput constant. This doubled range is explained by radio wave propagation physics, which dictate that, all other things being equal, a higher frequency signal will have a lesser range than a lower frequency signal. (Of course, this assumes a conservative 5 GHz path loss.)
Recently published papers based on varying models and techniques have compared the network capacity of 802.11a and 802.11b WLANs, but many reached contradictory conclusions. Here's why: one model can indicate that 802.11b networks offer superior performance to 802.1 la, but only under the assumption that all 802.1 la solutions transmit at 15 dBm, which isn't always the case. Another model can show clear network capacity advantages of 802.11a over 802.11b; however, the model is based upon a system that does not meet the full specifications of 802.11a, so the model does not provide a truly accurate representation of the full performance of optimized 802.11a systems.
Let's now assess the network capacity of wireless LANs by first looking at the network capacity offered by current 802.11a solutions and compare those to 802.11b solutions.
3COM TESTS 2.4 AND 5 GHZ PROPAGATION IN TYPICAL OFFICE ENVIRONMENT
The authors (Niels van Erven, Robert Yarbrough, and Lloyd Sarsoza) of a 3Com document entitled "2.4 and 5 GHz propagation measurements for WLAN," measured 2.4 and 5 GHz propagation in a common U.S. office environment. In other words, the test was conducted in a building with metal floor and metal roof, cubicle walls with metal sheets inside, and some drywall in the line-of-sight (LOS) path. In the case of a LOS path, where the Tx (transmit) and Rx (receiver) vertical polarized antennae were above the cubicles, the average path loss difference between 2.4 GHz (11b) and 5.2 GHz (11a) was around 7 dB. When the antennae had a non-LOS path (e.g., one antenna inside a cubicle), the difference was 2 to 3 dB.
The test results indicate that the more reflection there is in the signal's path, the less difference there is in propagation between the two frequencies. The test's results also suggest that with proper antenna positioning, 11b will have an additional link budget of about 6 to 7 dB compared to 11a. (However, for less optimal antenna positions, this difference is closer to 2 to 3 dB.)
It was also discovered that additional factors can play an important role in the actual range difference between 802.11b and 802.11a, e.g. the achievable Tx power (the transmit power of the radio) and Rx sensitivity (the receiver sensitivity of the radio), use of antenna diversity schemes, use of sophisticated time equalizers for 11b, etc. Current 802.11b implementations show a high level of optimization with respect to these parameters. (Note that for 802.11a, it is very difficult to get high Tx power with commercially available power amplifiers with linear performance to guarantee proper receiver sensitivity for the high bit rates.)
In another test, 3Com found that when 802.11b (running at 11 Mbps) and 802.11a (running at 6 Mbps) are compared, the data throughputs are similar. This is documented in a 3Com paper entitled "Comparing Performance of 802.11b and 802.1a Wireless Technologies," which states, "The available link budgets are also about the same." 3Com also found that the propagation is the only major difference. In fact, 3Com concluded, "In the best case, 802.11b can go roughly 50 to 70 percent farther than 802.11a. In the worst case the range is nearly the same." 3Com's overall conclusion based on its test was, "the high rate ranges of 802.11b are very similar to the 'low rate' ranges of 802.11a."
The importance of such tests is that they can help a network manager create a wireless network that can transition between 802.11b and 802.11a with respect to access point placement.
|