Abstract
Contents
- Introduction
- 1. Literature review
- 1.1 Gas ionization process
- 1.2 Features of plasma
- 1.3 Interaction of plasma with incident electromagnetic waves. Plasma frequency
- 2. Study of a plasma model
- 2.1 TThe Study of the different frequencies radio waves propagation through the plasma
- 2.2 The Study of the radio waves propagation through a plasma with a different electron’s collision frequency
- Conclusions
- References
Introduction
Research field of interaction of electromagnetic waves with a plasma medium is relevant for the fields of radiophysics and electronics. Plasma-based antennas have properties and features that are promising for many branches of radio engineering.
Plasma has charged particles, electrons and ions, therefore, it has conductivity. Plasma antennas can be used as antennas for transmitting and receiving radio signals. A plasma antenna is a device that has various advantages in the field of communication, the main advantage is that such an antenna can be turned off and on electrically. By changing operating parameters, for example, operating pressure, excitation frequency, input power, dielectric tube radius, plasma column length, gas material, a single plasma antenna can be converted into a matrix plasma antenna, spiral antennas. This demonstrates the possibility of reconfiguring plasma-based antennas. A change in the density and conductivity of the plasma changes the eigenfrequency of the plasma, so there is the possibility of reconfiguring one plasma antenna for different frequencies. When using a plasma antenna, it is possible to electronically control radiation in various directions [1].
1. Literature review
1.1 Gas ionization process
Unlike metals and electrolytes, gases are composed of electrically neutral molecules and atoms and do not have free charges (ions and electrons) that are capable of orderly movement under the influence of an electric field. That is, under normal conditions, gases cannot conduct electric current — they are dielectrics. However, the gas can also be a conductor if some of the molecules are split into electrons and positive ions. This splitting process is called gas ionization. Also, negative ions can appear in the gas due to the combination of part of the released electrons with neutral gas molecules.
Molecules and atoms of a gaseous medium can be represented as stable systems of charged particles. Therefore, for their ionization, it is necessary to do work against the forces of interaction of the ejected electron and the rest of the molecule (atom). This phenomenon is called ionization work. The magnitude of the work of ionization depends on the energy state of the electron pulled out of the atom, and also on the chemical nature of the gas. The weakest bond with the nucleus is in valence electrons, therefore, to tear out such an electron, less work is required than for any other. In this case, after the removal of one electron from the atom, the bond of the remaining electrons with the nucleus is strengthened. It follows from this that for pulling out each subsequent electron, it is necessary to do much more work than for the previous one.
The ionization work can be characterized using the ionization potential. The ionization potential is the potential difference that an electron must pass in an accelerating electric field in order for its energy to increase to the level of ionization work [2].
The gas can be ionized by various external influences. Possible effects include:
- strong gas heating;
- X-rays;
- α-, β-, γ-rays arising from radioactive decay;
- cosmic rays;
- bombardment of gas molecules by rapidly moving electrons or ions.
Quantitatively, the ionization process is characterized by the intensity of ionization, and is measured by the number of opposite signs charged particles pairs that occur per unit volume of gas per unit time.
Due to the fact that under normal conditions the gas is constantly exposed to radioactive radiation and cosmic rays, it can be concluded that the conductivity of the gas is never equal to zero, and it always has free charges, unless special measures are taken to protect the gas from any ionizing factors. Nevertheless, the intensity of ionization due to cosmic rays and the decay of radioactive elements scattered in the earth's crust is low, and therefore the gas conductivity, under normal conditions, although not zero, is very close to this value.
Gas ionization under the moving electrons and ions influence is called impact ionization. In the event of a collision of a particle with a neutral gas atom, it transfers part of its energy to it. If the kinetic energy of a particle is relatively small, then, based on experiments, its collision with an atom is in the form of an elastic impact. The energy that is transferred to the atom is insufficient for its ionization. It follows from this that the bombardment of gas atoms by low-energy particles causes only gas heating.
A completely different process occurs if a particle collides with an atom, the kinetic energy of which is large enough. Then the collisions are already inelastic and cause the excitation of gas atoms, that is, the atom goes from the normal energy level to a higher one, or if the energy supplied to the atom is large enough, then the atom is ionized. The minimum kinetic energy that a particle must have in order to effect impact ionization of a gas atom cannot be less than the ionization work and will be closer to it, the smaller the mass of the particle compared to the mass of the atom. For an electron, this energy is less than for any ion. For the implementation of impact ionization, ions need to pass a large potential difference in an accelerating electric field than electrons. The work that needs to be done to excite an atom is less than the work of ionization, therefore, inelastic collisions of particles with gas atoms can also occur if the particle has less energy.
For the processes of electrons and ions collision with molecules, which consist of two or more numbers of atoms, it must be taken into account that the excitation of such molecules can consist in increasing not only the energy of its electrons, but also the atomic nuclei oscillation energy and the energy of rotational motion of the molecule. Along with ionization, a process of ion recombination into neutral particles occurs in the gas volume [3].
1.2. Plasma features
In the nature of plasma, there are many features, and all of them are difficult to enumerate, however, some of the most important for the propagation of electromagnetic waves can be identified.
The first feature can be attributed to the fact that the values of the plasma parameters are very diverse, and the second feature is associated with this, often the plasma has significant heterogeneity. For a plasma, smooth, but significant changes in environmental parameters are typical. Such changes are sometimes so great that the dielectric constant changes sign [4].
Another feature of plasma is that, using plasma, it is possible without special effort to achieve a dielectric constant of zero or close to this value, as well as to provide weak absorption.
The fourth feature is a strong plasma frequency dispersion (permeability dependence of frequency).
The fifth feature is that the plasma parameters change strongly under the influence of a constant magnetic field. Thus, even weak magnetic fields significantly change the electromagnetic waves propagation nature in a plasma.
The sixth feature is that the electromagnetic properties of the plasma are nonlinear in relatively easily accessible fields. This leads to phenomena of nonlinear wave interaction that propagate in the plasma, in contrast to other substances, where nonlinearity of parameters can be achieved only in very strong fields [5].
1.3. Interaction of plasma with incident electromagnetic waves. Plasma frequency
Plasma from the electromagnetic properties point of view is an inhomogeneous, nonlinear and dispersive environment. The magnetic and dielectric constant and conductivity in a plasma can vary depending on the frequency and other parameters, which makes the plasma a special environment. As a result, for any incident wave frequency and any ionization density, there is one specific response. It is necessary to distinguish between the concept of plasma frequency and the working frequency of a plasma antenna. A plasma frequency is a measure of the amount of ionization in a plasma, and the working frequency of a plasma antenna is the same as the working frequency of a metal antenna [1].
Plasma ions and electrons oscillate with a certain frequency, which is called the plasma frequency. This frequency depends on the ions and electrons mass, and on the plasma density. The higher the plasma density, the higher its frequency. When an electromagnetic wave hits a plasma volume, the plasma responds in accordance with the ratio of the wave frequency to the plasma frequency. If the frequency of the electromagnetic wave is higher than the plasma frequency, the wave propagates through the plasma unhindered, otherwise the plasma reflects the wave as a conductive material.
2. Study of a plasma model
The study of the radio waves propagation through a plasma was carried out using computer electrodynamic modeling in the CST Studio Suite software. The principles of the program are described in [6, 7]. In the simulation software, a hydrogen-based plasma region was simulated with a concentration of free charges of 2,472 · 1017 m-3. The plasma frequency in this case is approximately 4.467 GHz [8]. Also, experiments were carried out on the radio waves propagation with a plasma at different electrons collision frequencies in it [9]. The plasma had a collision frequency of electrons in its volume equal to 2 · 105 collisions per second, 2 · 1010 collisions per second and 2 · 1020 collisions per second. Figure 1 shows a software plasma model with an electric field propagating from a radio waves source.
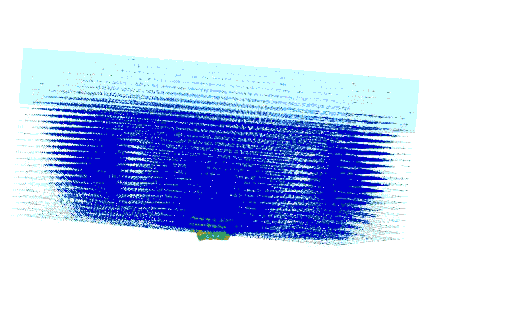
Figure 1 — Plasma model (natural plasma frequency: 2 GHz) with an electric field of the incident wave
(animation: 9 frames, 7 loops of repeating, 149 KB)
2.1 The Study of the different frequencies radio waves propagation through the plasma
The study was carried out by obtaining the pattern of the incident wave on the plasma region. First, two incident waves with a frequency of 1 and 4 GHz were simulated, which are less than the plasma frequency (Figure 2).
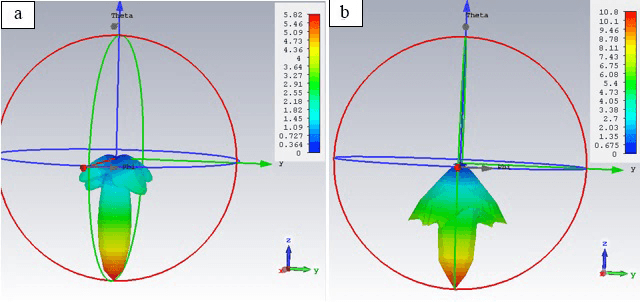
Figure 2 — The radiation pattern at an incident wave frequency: a) 1 GHz; b) 4 GHz
As can be seen from the results obtained, waves with frequencies lower than the plasma frequency are reflected from it, that is, the plasma in this case has properties similar to metal. Then, the case was simulated when the frequency of the incident wave is approximately equal to the frequency of the plasma. The simulation result is presented by the radiation pattern shown in figure 3.
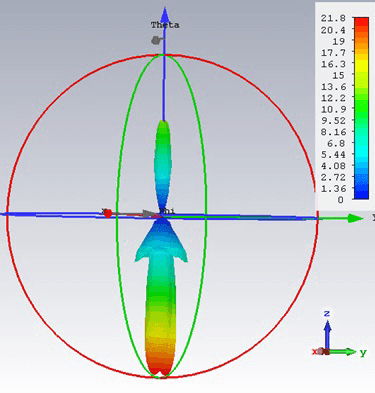
Figure 3 — The radiation pattern at an incident wave frequency of 4.5 GHz
Figure 3 shows that the wave is partially able to propagate through the plasma volume, however, a significant part of the energy is still reflected from it.
Next, the radio waves propagation with a frequency exceeding the frequency of the plasma was simulated. In figure 4 presents radiation patterns with frequencies of 5 and 10 GHz.
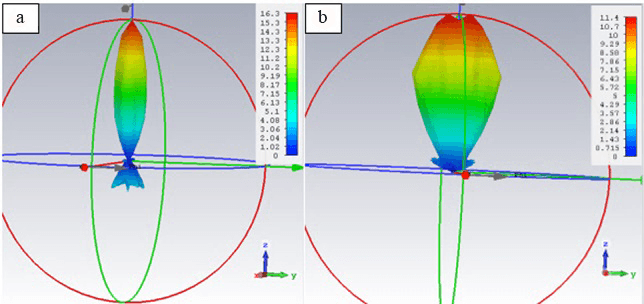
Figure 4 — The radiation pattern at an incident wave frequency: a) 5 GHz; b) 10 GHz
It can be seen from the obtained diagrams that when the frequency of the radio wave exceeds the frequency of the plasma, the plasma has almost no reflective effect. At a frequency slightly higher than the plasma frequency, an insignificant reflection of part of the energy of the incident wave is observed, and at a frequency noticeably higher than the plasma frequency, reflection is practically absent.
2.2 The Study of the radio waves propagation through a plasma with a different electron’s collision frequency
First, an experiment was conducted with a plasma whose frequency is higher than the radio wave frequency propagating through it. The signal frequency is 2 GHz. Figure 5 presents the radiation patterns formed as a software calculation result of the radio wave propagation through the plasma.
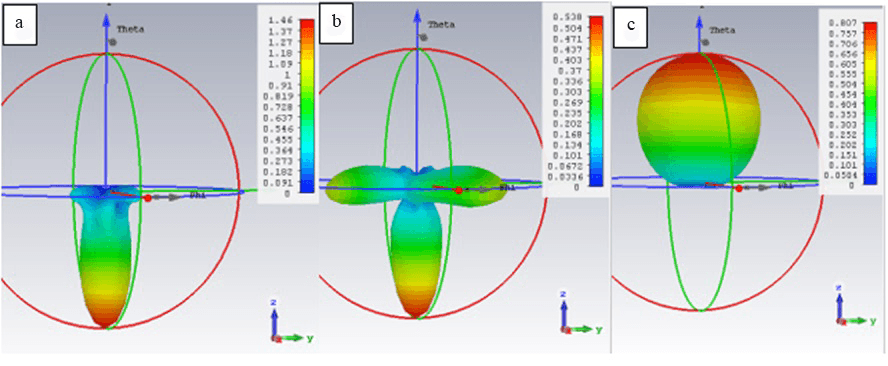
Figure 5 — The radiation pattern for a radio wave with a frequency of 2 GHz at an electron collision frequency: a) 2 · 105 collisions/s; b) 2 · 1010 collisions/s; c) 2 · 1020 collisions/s
Figure 5 shows that when the incident wave frequency is less than the plasma frequency, the signal energy is reflected, significant energy absorption and scattering in the plasma. At a lower frequency of electron collisions, the wave energy is largely absorbed and partially reflected from the plasma layer. With an increase, an increase in the degree of energy absorption and its dissipation in plasma is observed. At the highest electron collision frequency, the wave even partially propagates through the plasma volume.
At the next stage of research, the frequency of the radio signal was increased to 4.5 GHz, which approximately coincided with the plasma frequency. Figure 6 presents radiation patterns for this case.
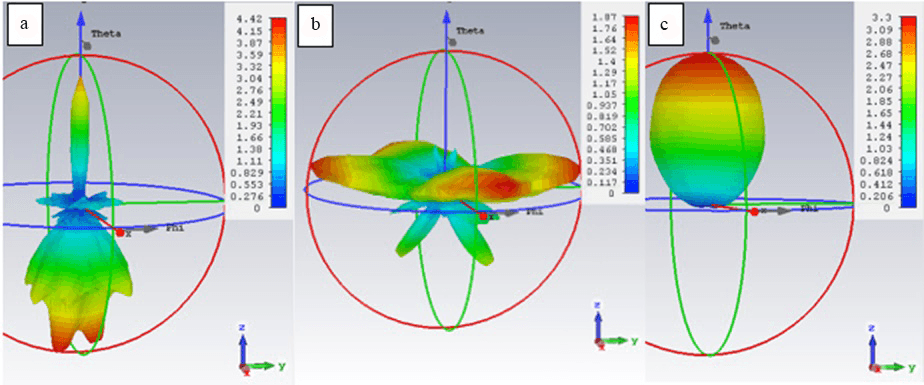
Figure 6 — The radiation pattern for a radio wave with a frequency of 4.5 GHz at an electron collision frequency: a) 2 · 105 collisions/s; b) 2 · 1010 collisions/s; c) 2 · 1020 collisions/s
Judging by the diagrams in figure 6 in the case when the frequency of the incident radio wave coincides with the frequency of the plasma, the signal experiences less attenuation than when the frequency of the wave is less than the frequency of the plasma. In this case, at a lower frequency of electron collisions in the plasma, the wave energy is absorbed, partially reflected, and some of the energy can overcome the plasma layer. With an increase in the collision frequency of electrons, the wave is significantly scattered in the plasma volume. With a further increase in the collision frequency of electrons, as in the case of the low frequency wave propagation, the wave passes through the plasma volume, however, in this case it experiences less absorption and scattering.
The last stage of this study is the study of the waves propagation with a frequency greater than the frequency of the plasma. Figure 7 shows the radiation patterns obtained in the software for a wave with a frequency of 10 GHz.
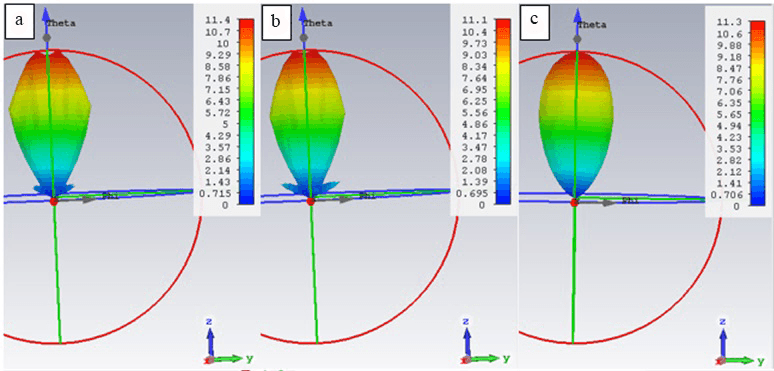
Figure 7 — The radiation pattern of a radio wave with a frequency of 10 GHz at an electron collision frequency: a) 2 · 105 collisions/s; b) 2 · 1010 collisions/s; c) 2 · 1020 collisions/s.
Judging by the figure 7, at a wave frequency exceeding the plasma frequency, the signal freely propagates through the plasma with almost no absorption, but at lower electron collision frequencies in the plasma layer there is a slight scattering of the wave energy. That is, the plasma in this case acts as a dielectric, without interfering with the electromagnetic waves propagation.
Conclusions
Thus, it can be seen that the plasma changes its properties for radio waves of different frequencies, reflects waves with a frequency lower than its own, and transmits waves exceeding its own frequency. And changing the plasma density, changing its frequency, it can be controlled the different frequencies waves propagation, or knowing the parameters of the plasma, calculate the frequencies necessary to overcome the plasma layer.
An increase in the electron collisions frequency in a plasma at the same charges oscillations frequency in it reduces the plasma ability to reflect electromagnetic waves. At the same time, the absorption processes and energy dissipation in space are amplified. If the frequency of the wave incident on the plasma layer is close to the plasma frequency, an increase in the electron collisions frequency leads to greater scattering and the signal propagation through the plasma volume. Moreover, the lower the wave frequency compared to the plasma frequency, the higher the energy absorption level, and an increase in the electron collision frequency leads to an even larger increase in absorption and enhances the energy dissipation in the plasma. If the signal frequency is higher than the plasma frequency, then a change in the parameter of the electron collision frequency no longer has a significant effect on the electromagnetic waves propagating through the plasma.
References
- Prince Kumar, Rajneesh Kumar,
Simulation of Plasma Antenna Parameters
, International Journal of Engineering Technology, Management and Applied Sciences, Vol. 4, Issue 5, ISSN 2349-4476, May 2016. - Физические величины: Справочник / А. П. Бабичев, Н. А. Бабушкина, А. М. Братковский [и др.].; Под. ред. И. С. Григорьева, Е. З. Мейлихова. — М.: Энергоатомиздат, 1991. — 1232 с.
- Курс физики (в трех томах). Т. II. Электричество и магнетизм: учеб. пособие для втузов / А. А. Детлаф [и др.]. — 4-е изд., перераб. — М.:
Высш. школа
, 1977. — 375 с. - Голант, В. Е. Основы физики плазмы / В. Е. Голант, А. П. Жилинский, И. Е. Сахаров. — М.: Атомиздат, 1977. — 384 с.
- Гинзбург, В. Л. Распространение электромагнитных волн в плазме / В. Л. Гинзбург. — М.: Наука, 1967. — 684 с.
- Курушин, А. А., Пластиков, А. Н. Проектирование СВЧ устройств в среде CST Microwave Studio. — М.: Издательство МЭИ, 2011, 155 с.
- Курушин, А. А. Школа проектирования СВЧ устройств в CST STUDIO SUITE. — М.: One-Book, 2014. — 433 с.
- Энговатов Д. С., Паслён В. В.
Особенности распространения электромагнитных волн в плазме
, XXI Міжнародна молодіжна науково-практична конференціяЛюдина і Космос
: Збірник тез — Дніпро: 2019, ISSN 2221-4550. - Энговатов Д. С., Паслён В. В.,
Исследование зависимости распространения радиоволн через плазму с различной частотой столкновения электронов
, Автоматизация технологических объектов и процессов. Поиск молодых: сборник научных трудов ХIХ международной научно-технической конференции аспирантов и студентов в г. Донецке 21–23 мая 2019 г. — Донецк: ДОННТУ, 2019.