Abstract
In the process of abstract writing the master's work hasn't been finished yet. Date of finish: December, 2012. The full thesis of master's work and materials according to the theme may be received from the author or his scientific advisor after the pointed date.
Content
- Introduction
- 1. Theme urgency
- 2. Goal and tasks of the research
- 3. The exclusion of blunders of the trajectory measurements results
- Conclusion
- References
Introduction
In the development and manufacture of flight, rocket and space technology is a large variety of complexity and nature of tests, such as laboratory, bench, and the most complex and expensive — flight. Modern aircraft (LA) are very complex and expensive systems. Requirements for them in terms of flight, economic, environmental and combat performance is constantly increasing. In this case, the role of practical tests to determine compliance with the parameters of a practical model of the theoretical. In the development of modern aircraft due to the impossibility of full and adequate theoretical description of up to half of all problems identified during testing. A long duration and high cost of their implementation are among the main factors of time and financial costs. Therefore, to solve the problem of cost reduction should improve the quality of practical tests, to improve the accuracy of the measuring systems and shoot as many as possible parameters for an experiment. Streamline the process of testing can be integrated in planning and handling the most accurate results.
1. Theme urgency
Due to technical and technological difficulties as well as high-value part of the aircraft, rocket and space technology to produce large quantities of objects for flight testing is impractical. This leads to a decrease in the number of experiments and significantly limits the ability to determine the effectiveness of the methods of statistical analysis of samples obtained during the experimental flight test data. Thus, there is a need to develop new combined analytical methods that take into account information about the object, previously accumulated in previous experiments and theoretical calculations and predictions. The reliability and completeness of the information depends on a priori the number of flight tests to determine the required performance.
Increased efficiency is achieved by measuring the most informative parameters or using a more precise measurement systems. In general, the movement of aircraft consists of the translational motion of the center of mass and rotational motion about the center of mass. The number of measured parameters are [1]:
- characterizing the position and movement of the center of mass with respect to different reference systems (coordinates of the object, its linear velocity and acceleration);
- determining the movement of the object relative to its center of mass (the corners of the course, pitch, attack);
- watching the operation of onboard systems (pressure, temperature, etc.);
- environmental parameters (pressure, humidity, etc.).
Trajectory measurements performs to determine the actual motion of the center of mass of the aircraft. In some cases, is to determine its angular orientation relative to the accepted system of coordinates [1]. Measuring systems can be optical (theodolite) and radio (radar). They may be located on land, ships or aircraft facilities. Radars have the advantage that they allow you to get much more options at long range than the optical, and to a lesser extent, dependent on weather conditions. But at the same optical methods for determining the parameters of the aircraft movements are more accurate. This is especially true is observed at very low altitudes near the ground, or at a small distance between the aircraft and radar.
2. Goal and tasks of the research
The aim of the research is to analyze the trajectory measurements data, anomaly detection and elimination of blunders, an algorithm for automatic processing of digital computing systems.
The main tasks of the research:
- Analysis of modern methods and means of trajectory measurements.
- Identifying the causes of hardware failure, the main parameters of accuracy and reliability of measuring instruments. The search for optimal methods to address these failures.
- The analysis of situations where there have been drastic changes in the nature of the movement of aircraft and the system makes a decision error. Finding ways to accommodate such events in the processing of measurement results.
Research object: trajectory measurements.
Research subject: the exclusion of gross errors (blunders) of the results of analysis of trajectory measurements.
3. The exclusion of blunders of the trajectory measurements results
The motion of the aircraft is quite a complicated process because it is at all times under the influence of external forces and depends on the parameters of the environment, construction, management and other factors. All this complicates the analysis, so the actual motion is replaced by a simplified model that reflects the basic laws of motion of the aircraft. The models are divided into dynamic and kinematic based on accounting information about the inertia of the aircraft and the forces acting on it during the flight.
The dynamical model is called deterministic if all existing forces are described by deterministic dependencies. Such models are used to describe the motion of artificial earth satellites (AES), parts of ballistic missiles and other aircraft in the area unmanaged (ballistic) flight. In this case it is assumed that the density of the atmosphere, air resistance and other external factors are known. In reality, it is not, therefore, such a model often fails to achieve the required accuracy of the movement of aircraft [2].
Dynamical model is used in determining the uncontrolled movements, performed under the action of the forces described by mathematical dependencies. Kinematic definitions for controlled movement of the aircraft. In the figure below presents the trajectories, described by the dynamic and kinematic models, ie, deterministic and nondeterministic trajectory.
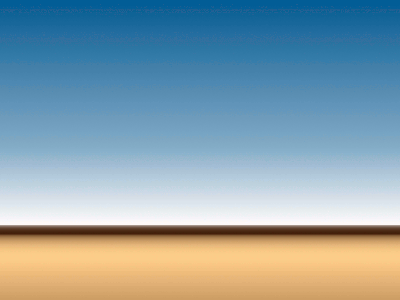
Figure 1 –
Deterministic and stochastic trajectories,
white — stochastic, yellow — deterministic
(animation: 8 frames, 300х400 px, 7 cycles, 31
kb, frame leight 500 ms, made with GIMP)
During the measurement, navigation parameters as a result of impact, a large number of random factors unrecorded errors of measurement. The presence of random errors in the measured parameters leads to a navigational error in the determination of surfaces and lines, and hence to errors in determining the coordinates of the aircraft. The value of the positioning error depends on the values of navigational error parameters and the methods of calculating the coordinates. The total error is considered to consist of three parts: a singular, regular and blunder.
Singular errors are a consequence of exposure to the measuring system of standing or slowly varying noise-factors (propagation conditions, inaccuracy of grading scales and linking to a system of universal time, etc.). Within one session of measurement errors are only marginally affected, so they are called systematic. They are defined as the difference between the arithmetic mean and the true value of the measured value:
Regular errors occur when the fluctuation deviations from normal conditions of measurement. For example, the deviations of the radio conditions, the drift of the operating frequency of the reference oscillator, the thermal noise of the equipment, etc. Such errors are called random, or rapidly changing. The distribution of the probability density of such errors due to the impact of a large number of random independent factor in most cases subject to the normal distribution law.
The third component — a gross error — caused by a sharp violation of the terms of the measuring system for the individual measurements. It is worth noting that sometimes a blunder made dramatic changes in behavior measured by a parameter that can sometimes occur in certain situations (for example, dropping a bomb leads to the relief of the aircraft and increasing its speed.)
Conclusion
Conduct external trajectory measurements and finding the best way to handle these results represent an actual problem. The cost and time-frames are sometimes up to half of all costs for the design of aircraft. Due to the greatly increased power of modern computers are becoming a popular statistical processing of measurement results, previously inaccessible because of the complexity of calculations. The proposed work methods can significantly improve the accuracy of measurement of the actual motion of the aircraft, eliminating or reducing the influence of various external factors, components of the error.
References
- Кринецкий Е.И.,
Александровская Л.Н., Мельников В.С., Максимов Н.А. / Основы испытаний
летательных аппаратов: Учебник для втузов / Под общ. ред. Е.И
Кринецкого. — М.: Машиностроение, 1989. — 312 с.:
ил.
ISBN 5-217-00355-3 - Жданюк Б.Ф. / Основы статистической обработки траекторных измерений. — М.: Сов. радио, 1978. — 384 с., ил.
- Harvey R.A. Probability, Approach to Aeronautical Research and Development / Aeronautical Jornal, 1973, 74, p 373 — 378/
- Аким М.А.,
Энеев Т.М. Определение параметров движения космических аппаратов по
данным траекторных измерений. —
Космические исследования
, 1963. / М. Минский. — М.: Мир, 1971. – 364 с. - Эльясберг П.Е.
Определение движения по результатам измерений. М.,
Наука
, 1976. - Кинг-Хили Д.
Теория орбит искусственных спутников Земли в атмосфере. Пер с англ.,
М.:
Мир
, 1966. - Раух Г.
Оптимальная оценка траекторий спутника с учётом случайных флюктуаций
лобового сопротивления. Пер с англ. —
Ракетная техника и космонавтика
, 1965, №4. - Агаджанов П.А.,
Дулевич В.Е., Коростелёв А.А.
Космические траекторные измерения. Радиотехнические методы измерений и
математическая обработка данных. М.:
Советское радио
, 1969. - Бахшиян Б.Ц.
Оптимальный выбор информации, используемой при определении траетории
движения космического аппарата. —
Космические исследования
, 1969. - Назаренко А.И.
Некоторые вопросы оптимизации обработки орбитальных измерений
искусственных спутников Земли. —
Космические исследования
, 1968. - Куликов С.М. — Авиация и ядерные испытания [Электронный ресурс]. — Режим доступа: http://militera.lib.ru/h/kulikov_sm/01.html.
- Михайлов М.В., Мотылев К.И., Щербов И.Л., Паслен В.В. — История возникновения и развития обобщённых методов и адаптивных алгоритмов обработки траекторной информации. — Матеріали IV Міжнародної науково-практичної конференції "Динаміка наукових досліджень — 2005". Том 25. Історія. — Дніпропетровськ: Наукаі освіта, 2005, с. 9 — 12.
- Румшинский
Л.З. Математическая обработка результатов эксперимента. Главная
редакция физико-математической литературы изд-ва
Наука
, 1971