Spectroscopy
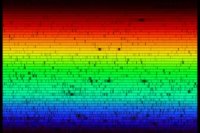
Extremely high resolution spectrum of the Sun showing thousands
of elemental absorption lines (fraunhofer lines) Spectroscopy is
the study of matter and its properties by investigating light,
sound, or particles that are emitted, absorbed or scattered by the
matter under investigation. Spectroscopy may also be defined as
the study of the interaction between light and matter. Historically,
spectroscopy referred to a branch of science in which visible light
was used for theoretical studies on the structure of matter and for
qualitative and quantitative analyses. Recently, however, the
definition has broadened as new techniques have been developed that
utilize not only visible light, but many other forms of
electromagnetic and non-electromagnetic radiation: microwaves,
radiowaves, x-rays, electrons, phonons (sound waves) and others.
Impedance spectroscopy is a study of frequency response in
alternating current. Spectroscopy is often used in physical and
analytical chemistry for the identification of substances through
the spectrum emitted from them or absorbed in them. A device for
recording a spectrum is a spectrometer. Spectroscopy can be
classified according to the physical quantity which is measured or
calculated or the measurement process. Spectroscopy is also
heavily used in astronomy and remote sensing. Most large telescopes
have spectrographs, which are used either to measure the chemical
composition and physical properties of astronomical objects or to
measure their velocities from the Doppler shift of spectral
lines.
Physical quantity measured
The type of
spectroscopy depends on the physical quantity measured. Normally,
the quantity that is measured is an amount or intensity of
something. - The intensity of emitted electromagnetic radiation
and the amount of absorbed electromagnetic radiation are studied by
electromagnetic spectroscopy (see also cross section). - The
amplitude of macroscopic vibrations is studied by acoustic
spectroscopy and dynamic mechanical spectroscopy. - Kinetic
energy of particles is studied by electron energy loss spectroscopy
and Auger electron spectroscopy (see also cross section). - The
mass-to-charge ratios of molecules and atoms are studied in mass
spectrometry, sometimes called mass spectroscopy. Mass spectrometry
is more of a measuring technique (metric) than an observation
(scopic) technique but can produce a spectrum of masses, a mass
spectrum, similar in appearance to other spectroscopy
techniques. - The number of molecules or atoms or
quantum-mechanical states to which the frequency or energy parameter
applies. In this case the spectrum is usually called cross
section.
Measurement process
Different types of
spectroscopy use different measurement processes: Absorption
spectroscopy uses the range of electromagnetic spectra in which a
substance absorbs. In atomic absorption spectroscopy, the sample is
atomized and then light of a particular frequency is passed through
the vapour. After calibration, the amount of absorption can be
related to the concentrations of various metal ions through the
Beer-Lambert law. The method can be automated and is widely used to
measure concentrations of ions such as sodium and calcium in blood.
Other types of spectroscopy may not require sample atomization. For
example, ultraviolet/visible (UV/ Vis) absorption spectroscopy is
most often performed on liquid samples to detect molecular content
and infrared (IR) spectroscopy is most often performed on liquid,
semi-liquid (paste,grease,and petroleum jelly), dried, or solid
samples to determine molecular information, including structural
information. Emission spectroscopy uses the range of
electromagnetic spectra in which a substance radiates. The substance
first absorbs energy and then radiates this energy as light. This
energy can be from a variety of sources, including collision (either
due to high temperatures or otherwise), and chemical
reactions. Scattering spectroscopy measures certain physical
properties by measuring the amount of light that a substance
scatters at certain wavelengths, incident angles, and polarization
angles. Scattering spectroscopy differs from emission spectroscopy
due to the fact that the scattering process is much faster than the
absorption/emission process. One of the most useful applications of
light scattering spectroscopy is Raman spectroscopy.
Common
types of spectroscopy

Fluorescence spectroscopy Fluorescence spectroscopy uses higher
energy photons to excite a sample, which will then emit lower energy
photons. This technique has become popular for its biochemical and
medical applications, and can be used for confocal microscopy,
fluorescence resonance energy transfer, and fluorescence lifetime
imaging.
X-ray spectroscopy and X-ray crystallography When
X-rays of sufficient frequency (energy) interact with a substance,
inner shell electrons in the atom are excited to outer empty
orbitals, or they may be removed completely, ionizing the atom. The
inner shell "hole" will then be filled by electrons from outer
orbitals. The energy available in this de-excitation process is
emitted as radiation (fluorescence) or will remove other less-bound
electrons from the atom (Auger effect). The absorption or emission
frequencies (energies) are characteristic of the specific atom. In
addition, for a specific atom small frequency (energy) variations
occur which are characteristic of the chemical bonding. With a
suitable apparatus, these characteristic X-ray frequencies or Auger
electron energies can be measured. X-ray absorption and emission
spectroscopy is used in chemistry and material sciences to determine
elemental composition and chemical bonding.
X-ray
crystallography is a scattering process; crystalline materials
scatter X-rays at well-defined angles. If the wavelength of the
incident X-rays is known, this allows calculation of the distances
between planes of atoms within the crystal. The intensities of the
scattered X-rays give information about the atomic positions and
allow the arrangement of the atoms within the crystal structure to
be calculated.
Flame Spectroscopy
Liquid solution
samples are aspirated into a burner or nebulizer/burner combination,
desolvated, atomized, and sometimes excited to a higher energy
electronic state. The use of a flame during analysis requires fuel
and oxidant, typically in the form of gases. Common fuel gases used
are acetylene (Ethyne) or hydrogen. Common oxidant gases used are
oxygen, air, or nitrous oxide. These methods are often capable of
analyzing metallic element analytes in the part per million,
billion, or possibly lower concentration ranges. Light detectors are
needed to detect light with the analysis information coming from the
flame.
- Atomic Emission Spectroscopy - This method uses
flame excitation; atoms are excited from the heat of the flame to
emit light. This method commonly uses a total consumption burner
with a round burning outlet. A higher temperature flame than atomic
absorption spectroscopy (AA) is typically used to produce excitation
of analyte atoms. Since analyte atoms are excited by the heat of the
flame, no special elemental lamps to shine into the flame are
needed. A high resolution polychromator can be used to produce an
emission intensity vs. wavelength spectrum over a range of
wavelengths showing multiple element excitation lines, meaning
multiple elements can be detected in one run. Alternatively, a
monochromator can be set at one wavelength to concentrate on
analysis of a single element at a certain emission line. Plasma
emission spectroscopy is a more modern version of this method. See
Flame emission spectroscopy for more details.
- Atomic
absorption spectroscopy (often called AA) - This method commonly
uses a pre-burner nebulizer (or nebulizing chamber) to create a
sample mist and a slot-shaped burner which gives a longer pathlength
flame. The temperature of the flame is low enough that the flame
itself does not excite sample atoms from their ground state. The
nebulizer and flame are used to desolvate and atomize the sample,
but the excitation of the analyte atoms is done by the use of lamps
shining through the flame at various wavelengths for each type of
analyte. In AA, the amount of light absorbed after going through the
flame determines the amount of analyte in the sample. A graphite
furnace for heating the sample to desolvate and atomize is commonly
used for greater sensitivity. The graphite furnace method can also
analyze some solid or slurry samples. Because of its good
sensitivity and selectivity, it is still a commonly used method of
analysis for certain trace elements in aqueous (and other liquid)
samples.
- Atomic Fluorescence Spectroscopy - This method
commonly uses a burner with a round burning outlet. The flame is
used to solvate and atomize the sample, but a lamp shines light at a
specific wavelength into the flame to excite the analyte atoms in
the flame. The atoms of certain elements can then fluoresce emitting
light in a different direction. The intensity of this fluorescing
light is used for quantifying the amount of analyte element in the
sample. A graphite furnace can also be used for atomic fluorescence
spectroscopy. This method is not as commonly used as atomic
absorption or plasma emission spectroscopy.
Plasma Emission
Spectroscopy In some ways similar to flame atomic emission
spectroscopy, it has largely replaced it.
- Direct-current
plasma (DCP) - Glow discharge-optical emission spectrometry
(GD-OES) - Inductively coupled plasma-atomic emission
spectrometry (ICP-AES) - Laser Induced Breakdown Spectroscopy
(LIBS) (LIBS), also called Laser-induced plasma spectrometry (LIPS)
- Microwave-induced plasma (MIP)
Spark or arc (emission)
spectroscopy - is used for the analysis of metallic elements in
solid samples. For non-conductive materials, a sample is ground with
graphite powder to make it conductive. In traditional arc
spectroscopy methods, a sample of the solid was commonly ground up
and destroyed during analysis. An electric arc or spark is passed
through the sample, heating the sample to a high temperature to
excite the atoms in it. The excited analyte atoms glow emitting
light at various wavelengths which could be detected by common
spectroscopic methods. Since the conditions producing the arc
emission typically are not controlled quantitatively, the analysis
for the elements is qualitative. Nowadays, the spark sources with
controlled discharges under an argon atmosphere allow that this
method can be considered eminently quantitative, and its use is
widely expanded worldwide through production control laboratories of
foundries and steel mills.
Visible spectroscopy
Many
atoms emit or absorb visible light. In order to obtain a fine line
spectrum, the atoms must be in a gas phase. This means that the
substance has to be vaporised. The spectrum is studied in absorption
or emission. Visible absorption spectoscopy is often combined with
UV absorption spectroscopy in UV/Vis
spectroscopy.
Ultraviolet spectroscopy
All atoms
absorb in the UV region because photons are energetic enough to
excite outer electrons. If the frequency is high enough,
photoionisation takes place. UV spectroscopy is also used in
quantifying protein and DNA concentration as well as the ratio of
protein to DNA concentration in a solution. Several amino acids
usually found in protein, such as tryptophan, absorb light in the
280nm range and DNA absorbs light in the 260nm range. For this
reason, the ratio of 260/280nm absorbance is a good general
indicator of the relative purity of a solution in terms of these two
macromolecules. Reasonable estimates of protein or DNA concentration
can also be made this way using Beer's law.
Infrared
spectroscopy
Infrared spectroscopy offers the possibility to
measure different types of interatomic bond vibrations at different
frequencies. Especially in organic chemistry the analysis of IR
absorption spectra shows what type of bonds are present in the
sample.
Thermal infrared spectroscopy
Thermal infrared
spectroscopy measures thermal radiation emitted from materials and
surfaces and is used to determine the type of bonds present in a
sample as well as their lattice environment. The techniques are
widely used by organic chemists, mineralogists, and planetary
scientists
Nuclear magnetic resonance
spectroscopy
Nuclear magnetic resonance spectroscopy analyzes
certain atomic nuclei to determine different local environments of
hydrogen, carbon, or other atoms in the molecule of an organic
compound or other compound. This is used to help determine the
structure of the compound. |