|
|
Хандильды Александр Васильевич |
Факультет:
компьютерных информационных технологий и автоматики |
Кафедра:
электронной техники
Специальность:
электронные системы |
Тема магистерской диссертации:
«Анализ технических параметров и средств построения электронной системы
промышленной беспроводной связи»
|
Руководитель:
Зори Анатолий Анатольевич,
д.т.н., проф.
|
|
ЭЛЕКТРОННАЯ БИБЛИОТЕКА
по теме:
«Анализ технических параметров и средств построения электронной системы промышленной беспроводной связи»
Автор: Andrew Miceli, Copyright © 2003 Artech House, INC. All rights reserved.
Название: Wireless Technician’s Handbook
Библиография: Artech House - ISBN 1-58053-357-4 - Norwood, 2003 - p.311
Оглавление
Избранные главы
|
|
Copyright © 2003 Artech House, INC. All rights reserved.
1.4 Understanding Propagation
|
The process of transmitting signals into the air is called signal propagation. Understanding
the concepts involved in taking a useful signal and sending it down a transmit
path to an antenna and out into the air is an essential aspect of a technician’s
position. A useful analogy to this process is a water hose. If we turn the faucet on for
a moment, we hope that all of the water will get to the nozzle and spray on our vegetable
garden. Of course, all of the water usually will not make it. A small leak in the
hose might send some of the water where we do not want it. A kink in the line might
slow the amount of water down. If it is a large kink, it could stop the water altogether,
and in fact send it back to the faucet (backwash), maybe causing more problems.
Of course, the best way to make sure everything works well is to use the right
diameter hose for the pressure you want.
RF is much the same (except that after a while of efficient watering, we do not end
up with plump tomatoes but rather happy customers). We generally have an amplifier,
our faucet, on which we attach a cable leading to the antenna, our nozzle. As we
send our signal down the cable to the antenna, we hope that all of the power makes it
through the route and out the antenna, but there are reasons why this often does not
occur: a bad cable that isn’t shielded enough, a connector that is not tightened nearly
enough, some sort of break in the transmission line, or a flaw in the antenna.
One advantage in the RF world is that by using what is called an RF bridge, we
can separate what we are transmitting down the cable and what is coming back
the other way (the reflected power—the power that did not make it out shown in
Figure 1.7). Chapters 10 to 14 will give more detail on these testing methods.
What also is important to understand is that different frequencies propagate differently.
Generally, lower frequencies will travel much greater distances, but they
are also more susceptible to physical obstacles, such as a wall. Also, lower frequencies
will propagate with a much wider footprint than will higher frequencies; in
other words, higher frequencies have more propagation loss than lower frequencies.
Higher frequencies are much more directional (hence the use of microwave signals
for line-of-sight point-to-point transmission systems). Thus, transmitting 50W at
570 kHz is quite different than transmitting 50W at 800 MHz. In cellular systems,
we take advantage of this directionality when we divide cells into sectors. More on
this appears in Chapter 2.
Figure 1.7 Reflected power is a good indicator of antenna and transmit path performance.
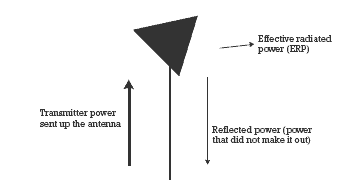
The concept of fading also needs to be understood. Fading generally refers to
the degradation of the signal at the receiver. There are four main fading effects
related to physical conditions:
• Attenuation due to distance;
• Attenuation due to environmental features;
• Raleigh fading;
• Multipath fading.
Naturally, as a receiver moves away from the transmitter or places large objects
between itself and the transmitter, the signal level will decrease and lower the call
quality. When the subscriber moves a significant distance away from the transmitter,
the fading that occurs is called large-scale path loss, as the attenuation is due to
the distance. Shadowing generally refers to a type of fading that occurs when
the subscriber moves behind a large object or perhaps in a tunnel or the like (see
Figure 1.8). These two fades are naturally an obstacle to all wireless systems,
although some systems are able to overcome the problem easier than others.
Multipath fading is another type of fading caused by interference between two
or more waves leaving the transmitter at the same time, but taking different paths to
the receiver and thus arriving at the receiver at different times. This can cause the
signals to have different phases as they enter the receiver, and this will cause a
destructive process, lowering the actual received level. It should be understood that
the multipaths can arrive in phase, and thus they would add constructively—a
sought-after condition.
Shadowing, a sudden drop or gain in signal strength, occurs when subscribers pass behind structures
Figure 1.8 Fading can be caused by a variety of circumstances, such as distance from the transmitter
or shadowing from large objects like mountains.
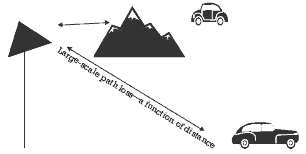
If the multipaths could some how be demodulated separately, and then combined
coherently, this would substantially improve the system. This is what cdma-
One does with its Rake receiver. Problems in digital systems related to signals taking
different paths to the receiver, and thus arriving at different delays, are also often
called intersymbol interference (see Figure 1.9).
Fading related to the motion of the terminals (i.e., driving in a car) is often called
Rayleigh fading. Because of the movement of the handset, the received signal rays
undergo what is known as a Doppler shift. This causes a shift in the wavelength of
the signal, and like the multipath problem described earlier, causes multiple signals
at different phases to enter the receiver and degrade (or sometimes improve) reception. The nature of this type of fading causes the changes to occur very quickly,
and sometimes this type of fading is called fast fading.
Figure 1.9 Signals can take different paths to the receiver. These multipaths thus take different
amounts of time to get to the receiver and can cause problems at the receiver.
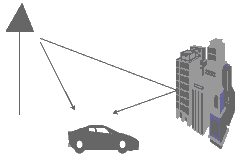
Fading can either be frequency selective, acting much like a notch at a specific
frequency, perhaps as wide as 300 kHz, or the fades can be flat, causing a problem
across a very large bandwidth. One of the best defenses against deep fading is to
widen the bandwidth of the signal. Considering that fading can often be frequency
selective, one can easily understand how a system that uses a wider frequency band
will be able to withstand a fading environment better than a narrowband system
(see Figure 1.10). This is one of the main reasons that spread spectrum systems are
becoming popular. Also, the fact that fades can be frequency selective explains why
the mobiles transmission to the base station may be terrible, but yet the base station
connection to the mobile is fine, as these two channels will operate on different frequency
bands (separated by either 45 MHz or 80 MHz). These concepts will be discussed
in detail later in the book.
|
1.5 The Transmit and Receive Path
|
A logical way of looking at a transceiver is to divide the modulation section from the
transmit path section. In other words, we can examine the system that modulates
and demodulates the information separate from the section that takes the modulated
signal and prepares it to be sent out on the air (in the case of the transmitter),
and the section that receives the signal off the air and prepares it to be demodulated
(in the case of the receiver). The former section will be discussed later in this chapter,
as modulation types are discussed. The latter, which we will refer to as the transmit
and receive path section, includes connections and cabling from the modulators tothe amplifiers and filters, the connectors and cabling to the antenna, and the antenna
itself.
Figure 1.10 A deep fade can occur in a specific frequency band (shown by the black fill). Naturally,
the wider the bandwidth of the desired signal, the more resilient it is to these deep fades.
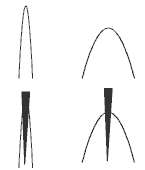
Figure 1.11 In today’s high-tier wireless systems, uplink and downlink channels are separated by
frequency (frequency-division duplex).
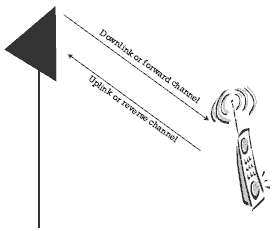
In the main high-tier wireless systems in use today, the mobile transmits on one
set of frequencies and the base station transmits on a different set of frequencies.
This setup allows for full duplex communications. The mobile transmit band is
known as the uplink, or reverse channel. The base station transmit band is known as
the downlink, or forward channel (see Figure 1.11).
Filters are self-explanatory. They basically clean up a signal, ensuring that any
unwanted power outside of the transmit or receive bandwidth is attenuated. Obviously,
filters are frequency dependent, and are generally labeled lowpass, highpass,
or selective (and sometimes selectable). These are indications of which frequencies
they will allow to pass. As filters are usually passive devices, they do not fail very
often in the field, although they can degrade in performance over time. Filters are
generally tested by using scalar network analysis (discussed in Chapter 10), which
involves sweeping a generator across frequencies at the input and measuring the frequency
response at the output (see Figure 1.12).
Amplifiers are used to boost power to a level needed to communicate with other
transceivers and to boost received power (these are often called preamplifiers) so
that the information can be detected and demodulated. Amplifiers that boost power
in terms of watts are known as power amplifiers (PAs). Amplifiers come in many
shapes and sizes, and they are one of the primary failure areas of transceivers. This is
because they are active devices and generally produce substantial amounts of heat in
the presence of high-current circuitry.
Figure 1.12 Swept frequency analysis is used to measure RF devices in a frequency domain. Here,
we see the transmit characteristic of a lowpass filter (note that the higher frequencies are attenuated).
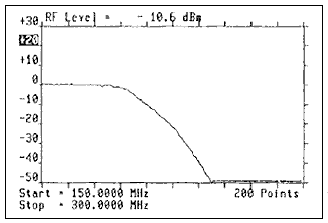
While amplifier designers try to make their products as linear (frequency independent)
as possible, most amplifiers are still nonlinear devices. When RF signals
are passed through nonlinear devices, the possibility exists for a form of mixing that
causes distortion—more specifically, intermodulation distortion—which can result
in poor signal quality or mathematically related interference products at the output
of the amplifier. Harmonics (which occur at multiples of the carrier) can
also increase or develop in amplifiers, particularly when the signal is overdriven (see
Figure 1.13).
|
1.6 Digital Basics
|
|
It may seem out of place to jump from RF propagation to a review of digital technology,
but today’s complex communication systems are a blend of digital and RF. In
fact, the RF portion of these new standards is really the last step in a many-step
process of getting the information transmitted. Before we can begin to understand
digital modulation, it is important to understand the form of the information we are
modulating.
Figure 1.13 Harmonics and intermodulation. Products are usually undesired results of bad
devices and are mathematically related to the fundamental frequency.
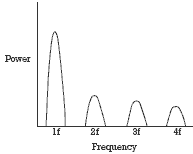
When we speak of digital, what we are really talking about is the use of ones and
zeros to represent data and perform actions. For instance, a computer can use a
series of ones and zeros to represent a particular letter of the alphabet, which then
allows the computer to store the sequence electronically. It is important to understand
that computers speak in digital, and even the “analog” first generation cellular
phone system needs the ability to transmit digital information (ones and zeros) in
order to relay signaling information.
Usually, this is done by using voltage levels to represent the ones and zeros.
Thus, a high voltage might represent a one, and a low voltage might represent a zero.
Often, a bipolar digital system is used, in which a high is +1V, and a low is –1V. This
allows some easier mathematical manipulation and is generally used in wireless
applications.
You can begin to see that ones and zeros could really be represented by any
method that has two easily recognizable states—high and low voltage is but one way
to perform this task. For instance, we can shift frequency over set periods of
time—one frequency for a moment for a one, then another if we want to represent a
zero. Another way would be to change the amplitude of the signal depending on the
bit to send, perhaps turning the amplitude on for a one and down for a zero. Naturally,
some sort of clocking system would need to be in place for this to work.
We could also shift the phase of a signal—one phase represents a one, while
another represents a zero. Additionally, we can use relative changes as opposed to
absolute positions. For instance, a change of –45° of phase might represent a one
and a change of +45° might indicate a zero. This is how we will represent ones and
zeros on the RF carrier, which will be discussed later. This form of shifting from one
state to another to represent ones and zeros is known as shift keying.
Thus, we have frequency shift keying (FSK), phase shift keying (PSK), amplitude
shift keying (ASK), and a combination of any two in some systems (see Figure 1.14).
Figure 1.14 Keying certain characteristics to represent ones and zeros.
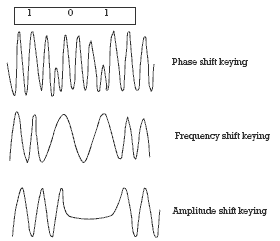
Up to the point where the data is actually modulated on the final RF carrier that
will be sent out on the air, data is manipulated in standard high or true and low or
false voltage digital levels. It is important to review some of the basic digital functions
in order to understand how digital sequences are manipulated.
Several logic gates are common in cellular applications (see Figure 1.15):
• AND gate: In the AND gate, two trues at the inputs will result in a true output.
Any false on an input will result in a false output.
• OR gate: In the OR gate, any true value on an input will result in a true out
put. The only time it will output a false is if both inputs are false.
• Exclusive OR (X-OR) gate: The X-OR gate is very important in digital systems,
particularly spread-spectrum systems. The X-OR gate is used to spread
sequences (this will be discussed later). A true is output when opposite values
are input. In other words, when the input is a false and a true, the output will
be true.
• Inverter gate: Naturally, the output will be the inverse of the input.
• Shift register: A shift register is a logic device used to store a binary value. As it
is toggled, it moves its stored value to the output.
Another concept that is important to understand is digital correlation. Correlation
is the amount of similarity between two items. In this case, it is an actual measurement of the similarity between two digital sequences.
Figure 1.15 Logic gates common in cellular applications.
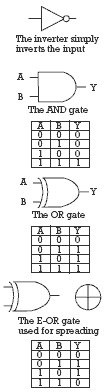
If you look at Figure
1.16, you can see how we objectively measure digital correlation. By multiplying the
bipolar values, we will end up with a digital waveform product. If we integrate (or
find the area of) this resulting waveform, we have a correlation measurement. If
100% of the area is present, it would indicate perfect correlation, or a one. Perfectly
uncorrelated signals would be measured as zero. If two digital signals are perfectly
uncorrelated, it can also be said that they are orthogonal to each other. You may
have heard of Walsh codes in code-division multiple access (CDMA). These Walsh
codes are actually orthogonal sequences.
Figure 1.16 The product of two perfectly correlated signals will have an area of one. Any difference
between the two signals would result in an area less than one.
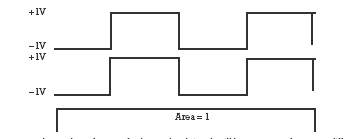
|
|
Хандильды А.В. Designed for Портал магистров ДонНТУ
|
|