Abstract
Content
- Introduction
- 1. Theme urgency
- 2. Goal and tasks of the research
- 3. Literature review of publications on the carbon dioxide solubility in organic absorbents
- 3.1 Current status of the investigation of carbon dioxide solubility in organic absorbents
- 3.2 Methods of carbon dioxide solubility investigation at elevated pressure
- 4. Solubility measurement technique of carbon dioxide in organic absorbents at elevated pressure
- Conclusion
- References
Introduction
Natural gas contains significant amount of impurities, the main of which are carbon dioxide, hydrogen sulfide, mercaptans, water vapor, etc. Concentration of these components can be rather high. For example in the Astrakhan natural gas the carbon dioxide content is 26%, and hydrogen sulfide is 16% [1]. Herewith the Astrakhan gas deposit is the largest deposit in Europe.
In the process of extraction and transportation of natural gas, especially at low temperatures, the formation of gas hydrates – clathrate compounds of gas components and water (snowlike masses) can take place. Gas hydrates may block the cross-section of pipelines, disturb their normal work and even may damage them. Furthermore, the gas purification from the undesirable impurities reduces cost of transportation and reduces the corrosive effect of the communications.
Most of the used in chemical industry process gases contain carbon dioxide, water vapor and hydrogen sulfide. For example, the gas for ammonia synthesis obtained by high-temperature conversion of natural gas contains 23-24% of carbon dioxide [2]. Since the impurities in the syngas leads to decrease in the concentration of the main component, corrosion of equipment, catalyst poisoning, it is necessary to remove them.
Due to the large amounts of natural and process gases used in the chemical industry it is effective to extract carbon dioxide, hydrogen sulfide and water vapor by organic physical absorbents. Effectiveness of such solvents strongly depends on partial pressure of the extracted component. For the regeneration of the saturated absorbent from the most part of the absorbed volatile component the process of depressurization is sufficient. Widely used as moisture absorbents are diethylene glycol (DEG) and triethylene glycol (TEG). Due to high viscosity of glycols at low temperatures it is appropriate to add another component in order to reduce their viscosity. Such a component, in particular, can be N-methylpyrrolidone (NMP), which has a low viscosity and well absorbs moisture. Mixtures DEG with NMP can be used for gas drying at temperatures up to -20°C, which significantly improves the quality of the dried gas. Simultaneously undesirable components such as hydrogen sulfide, mercaptans and carbon dioxide are removed from the gas.
Plant designing and creation of technological schemes for purification of gases from carbon dioxide with the help of organic absorbent requires data on the parameters of liquid-vapor equilibrium in the system carbon dioxide – absorbent at high pressures and knowledge of the phase diagram of the system. The lack of such a data in the scientific literature demands experimental determination of carbon dioxide solubility in absorbent DEG – NMP at elevated pressures.
1. Theme urgency
Existing methods of gas purification from impurities are imperfect. Advantageously currently used chemical absorption techniques allow to hold deep scrubbing of impurities. Purification by chemical absorption is very costly for large volumes of treated gas and high content of impurities in the gas composition. It is appropriate to use the phenomenon of physical absorption for the process of purification. This will enable us to reduce the cost of purification process and improve it's efficiency.
2. Goal and tasks of the research
The aim of the investigations is to develop a process for purification of gas mixtures from carbon dioxide with the help of organic absorbent based on the determination of carbon dioxide solubility by the absorbent DEG – NMP at elevated pressures.
Main tasks of the research:
- Determination of the carbon dioxide solubility in mixtures DEG – NMP.
- Calculation thermodynamic parameters for carbon dioxide in the absorbent DEG – NMP.
- The development of the technological scheme for gas purification from carbon dioxide and determination the scheme optimal work parameters.
Planned following actual scientific results:
- The solubility data of carbon dioxide in absorbent DEG – NMP with different ratios of components.
- The basic thermodynamic parameters of carbon dioxide dissolution in the absorbent.
- Diagram liquid-vapor equilibrium in the system carbon dioxide – DEG – NMP.
- Basic purification parameters of gas mixtures of carbon dioxide, hydrogen sulfide and water vapor using absorbent DEG – NMP.
3. Literature review of publications on the solubility of carbon dioxide in organic absorbent
3.1 Current status of the investigation on carbon dioxide solubility in organic absorbents
Over the past few decades in the scientific literature there is a tendency to an increase in the number of published studies on the liquid-gas systems containing carbon dioxide. For example, out of all the investigated in the last 5 years two-component liquid- gas systems at elevated pressure, 54% of them contained carbon dioxide as one of the components, for the three-component systems this figure is even higher – 66% [3].
Such amount of researches due to the fact that the scope of the carbon dioxide is continuously expanding. It mainly used as supercritical solvent in extraction of various organic compounds. Carbon dioxide has the following advantages over other supercritical solvents: cheapness, non-toxicity, non-flammability, ease of obtaining a pure, low critical temperature (which prevents decomposition of extractives) and ease of separation of the component solution by releasing the pressure and evaporating carbon dioxide.
N-methylpyrrolidone is a good absorbent for carbon dioxide removal in a reformed gas producing hydrogen by steam-oxygen conversion of hydrocarbons under pressure and the gas produced methane conversion in tubular furnaces. After conversion of carbon monoxide the gas is compressed to 7,6-13,7 MPa and is purified with the help of NMP [4]. Advantages of NMP: absence corrosive properties, non-toxicity and easy biodegradability in case of contact with sewage [5].
The basic physical and chemical properties of NMP [6]:
Тable 1 – The basic phisycal and chemical properties of NMP
The empirical formula | C5H9ON |
The molecular weight, g/mol | 99,13 |
Boiling temperature, °С | 205 |
Melting temperature, °С | –24,2 |
Density at 20°С, g/сm³ | 1,033 |
Refraction index n20 | 1,4709 |
Viscosity at 20°С, mPa⋅s | 1,9 |
Vapour pressure at 40,5°С, mm. Hg. col. | 1,0 |
Diethylene glycol – a colorless or yellowish clear liquid. Basic physical and chemical properties of it are listed below [7]:
Тable 2 – The basic phisycal and chemical properties of DEG
The empirical formula | C4H8O(ОН)2 |
The molecular weight, g/mol | 106,12 |
Boiling temperature, °С | 244,8 |
Melting temperature, °С | –7,8 |
Density at 20°С, g/сm³ | 1,1161 |
Refraction index n20 | 1,4472 |
Viscosity at 20°С, mPa⋅s | 35,7 |
Vapour pressure at 20°С, Pa | <1,3 |
Solubility of carbon dioxide in DEG with temperature depending at pressures 1,5,10,20 atm at given in the work [8]. Solubility isotherms of carbon dioxide in DEG are shown in Figure 1[9].
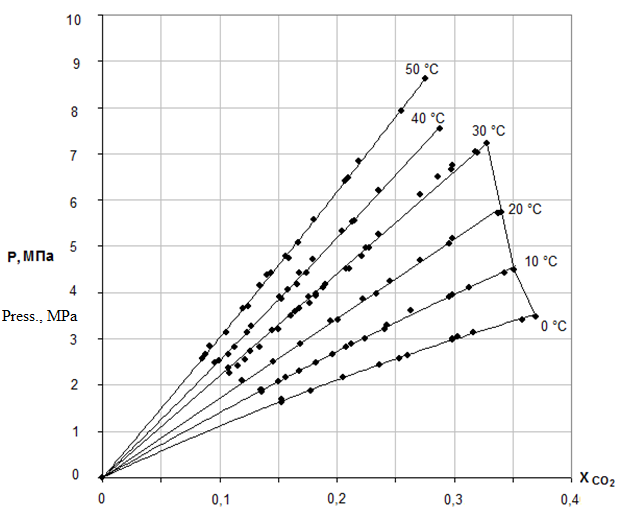
Figure 1 – Solubility isoterms of carbon dioxide in DEG
Liquid-gas systems, containing carbon dioxide and another component – DEG or NMP are investigated in the works [3,10-14]. However, only a relatively small number of works is devoted to the study of systems of interest: with absorbents – DEG, TEG, NMP. The data available in the scientific literature, often are far from complete. In the scientific literature data for solubility in these systems were obtained only in a small range of temperatures and pressures. In the work [12] range of temperature is 298-398 K, the range of pressures is 0-21,1 MPa. In the work [13] the temperature was varied from 298 to 318 K, the pressure from 0.9 to 8.4 MPa. In the work [15] measurements were carried out at a temperature of 313 K in the pressure range from 0.72 to 7.78 MPa. It should be noted that data of various authors significantly differ among themselves.
System of carbon dioxide – DEG – γ-BL was investigated in the work [16]. γ-BL and NMP have similar structure and physico-chemical properties, so one should expect the similar phase behavior of the system carbon dioxide – DEG – NMP. All of the mentioned above is the basis for generalization and systematization of available information.
3.2 Methods of carbon dioxide solubility investigation at elevated pressure
Phase equilibria Investigation methods can be divided into two large groups: analytical and synthetic methods [17]. The main difference between these methods is in the method of determining the composition of the equilibrium phases. In the analytical methods of research part of phase of the system selects and exposed the analysis of the sample by analytical chemistry methods. In the synthetic methods phase composition is determined by indirect measurements in the system of a given composition.
As an example of the synthetic method phase equilibria investigation in the liquid-gas system can be work [15]. Research installation used in this study is shown in Figure 2.
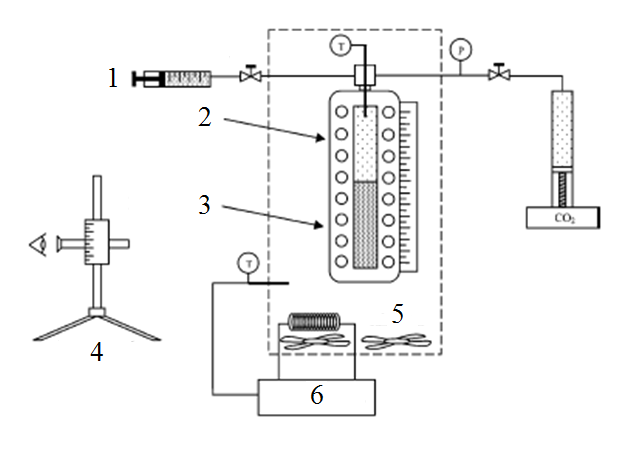
Figure 2 – Research installation for phase equilibria by synthetic method
1 – liquid components, 2 – vapor phase, 3 – liquid phase, 4 – cathetometer, 5 – air bath, 6 – temperature controller
The working volume of the cell is 150 cm³, which was measured by adding a known amount of gas to the cell at constant temperature and measuring the resulting pressure. The volume scale on the sight gauge was calibrated by adding known volumes of water and measuring the resulting height using a cathetometer. The equilibrium cell was placed in a temperature-controlled air bath. The temperature of the air bath and vapor phase inside the cell was monitored with a thermocouple and digital readout. The air bath temperature was maintained by a digital temperature controller. The cell is mounted on a rotating shaft, and mixing is achieved by rotating the entire cell.
As the synthetic methods is not accompanied by sampling the composition of the system remains unchanged. This technique has the following drawbacks: complexity of installation, a large volume of equilibrium cell, requiring significant reagent consumption, for each possible load holding only one measurement at a given temperature.
Syntethic measurement method was also used in work [18]. Installation scheme is shown in Figure 3.
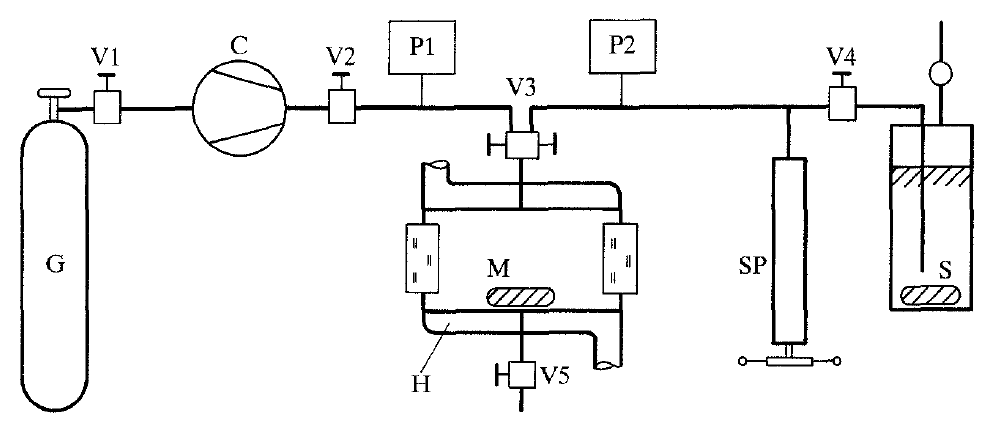
Figure 3 – Schematic diagram of the apparatus for the synthetic method of measurement
G – gas storage, С – diaphragm compressor, М – high-pressure optical cell, Н – heating jacket, SP – high-pressure
screw press, S – methanol storage
The heart of the apparatus is the high-pressure optical cell M. Depending on the mole fraction of the methanol were used two cells of different sizes: 30 cm³ capacity, maximum operating pressure 200 MPa at 600 K and 110 cm³ capacity, maximum operating pressure 70 MPa at 600 K. The temperature of the cell M was controlled by a circulatory thermostat connected to the welded heating jacket H of the cell. The diaphragm compressor C transferred pure gas from the gas storage G into the cell M. The mass of gas thus introduced into the cell was calculated from the measured values for the pressure and temperature and the known volume of the cell by means of a reliable equation of state. The one exception was metering carbon dioxide for the 298.15 K isotherm. Since it's critical temperature is high, uiz. 304.2 K, the carbon dioxide was metered at 273.15 K with a screw press. It's temperature was controlled by a thermostat, and the volume of carbon dioxide metered was determined at a pressure of 7.0 MPa in each case. A density was taken to calculate the mass of carbon dioxide. The methanol was added with the aid of the high-pressure screw press. The pressures were measured by means of different strain-gauge pressure transducers having suitable measuring ranges. The pressure of the pure gas was measured by transducers. It was estimated that the total error in pressure measurement is less than 0.002%. Maximal error of temperature measurement is 0,5 K.
The main advantage of the analytical investigation methods is the ability to determine the equilibrium phase composition by methods of analytical chemistry. This method has the following drawbacks: complexity of the equipment design, the need for significant amounts of reagents, long enough time to establish equilibrium, and thus considerable time for carrying out measurements. Sampling for analysis affects the entire system, resulting in errors in determining the composition of the sample which may differ from that of the entire system composition. Consequently, for the same error in the received data over attractive are the synthetic methods of investigation of phase equilibria.
The techniques described above have a number of disadvantages which do not allow us to use them in performing this work. Therefore to determine solubility of carbon dioxide in the DEG – NMP mixtures technique used in the work [19] was chosen.
As it was already mentioned, the liquid system DEG – NMP was recommended as an effective low-temperature gas dehumidifier. This fact is based on the high absorbent capacity of the system relative to the water vapor, low temperature of crystallization and its low viscosity at low temperatures. In order to use this system as a carbon dioxide absorbent it is necessary to determine solubility of carbon dioxide in the proposed solvent.
4. Solubility measurement technique of carbon dioxide in organic absorbents at elevated pressure
Installation used for investigation of liquid-gas equilibrium at elevated pressures is given in Figure 4
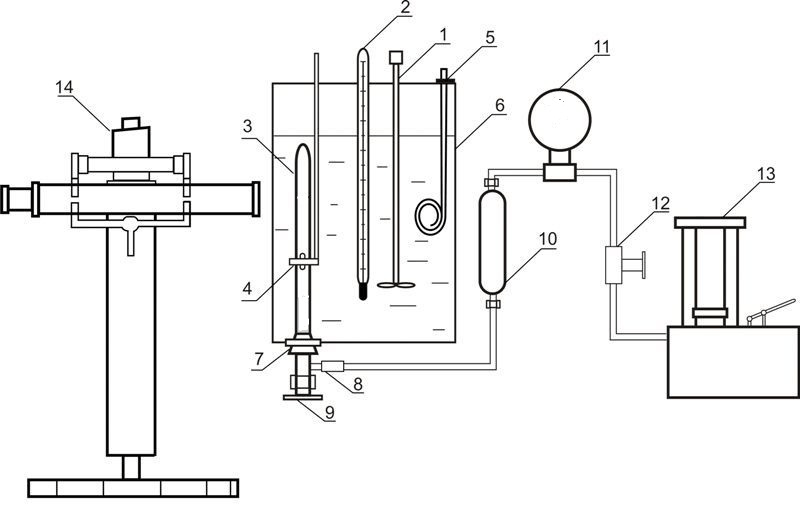
Figure 4 – Installation for investigation of liquid-gas equilibrium at elevated pressures 1 – agitator; 2 – thermometer; 3 – equilibrium cell; 4 – magnet; 5 – heater; 6 – thermostate; 7 – rubber cork; 8 – releasable contact; 9 – valve; 10 – oil-mercury separator; 11 – standard thermometer; 12 – valve; 13 – oil press; 14 – cathetometer
The installation consists of equilibrium cell made out of transparent quartz glass 3, the content of which is compressed with the help of mercury and is mixed with nickel stirrer, which is inside the cell. Movement of the agitator is due to axial movement of the outer annular magnet 4. Pressure in the cell is determined by standard manometer 11, the correction is introduced by mercury column height difference in the cell and the separator 10. Mass of the cell is about 60 grams, so the weight of the components was determined by weighing on an analytical balance. After loading the absorbent removal of dissolved air in it was carried out by vacuuming. The carbon dioxide was loaded in the cell by freezing in liquid nitrogen.
The temperature is maintained by a thermostat 6 and measured by normal thermometer 2. Pressure measurements are performed by standard manometer 11. Phase column heights in the cell are measured by cathetometer V-630.
To establish the state of equilibrium cell the contents mixed by agitator.
Equilibrium cell is shown in Figure 5.
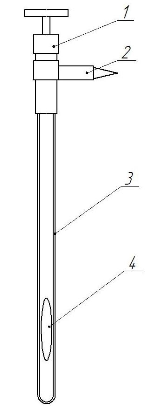
Figure 5 – Equilibrium cell
1 – valve; 2 – releasable tapered contact; 3 – cell ampoule; 4 – agitator
It is necessary to calibrate the cell before the use of it for phase equilibrium investigations. Calibration was carried out with the help of degassed distilled water. The gases were removed by boiling water and cooling it to room temperature without air access. Holding calibration allows us to obtain an equation describing the volume dependence of the phase height in the cell.
The cell is vacuumed and weighed on an analytical balance. Thereafter from the valve unscrewed stud and via medical syringe loaded with the solvent mixture. The stud is screwed, the solution is frozen by placing the cell in liquid nitrogen and the cell is vacuumed. When it is detached from the vacuum line the solvent is melting. After this, the cell is again immersed in liquid nitrogen and vacuumed. For the complete removal of the air dissolved in the liquid is necessary to repeat this operation 5-6 times. Cell with a degassed solvent is weighed and the difference in weight of the loaded and empty cell gives the mass of the component.
After addition of the solvent the loaded cell is connected to the vessel of known volume containing carbon dioxide. From the contact line, after the opening of the cell and it's immersion in liquid nitrogen carbon dioxide is frozen. Then the cell is closed, warmed and weighed.
The loaded cell with the help of a rubber tube is inserted into the bottom of the thermostat 6 and is connected by conical connection 8 to the mercury-oil separator 10. Capillar of the separator top is disconnected from the oil line and joined through the separation volume to the oil pump. Then removal of air from the connection lines takes place. After disconnecting the vacuum pump mercury fills the line from separator line 10 to the valve seat 9. Oil-mercury separator is joined to the oil line by tapered contact and pressure is raised using oil press 13.
Before the opening of the cell valve pressure in the line must be raised higher than the equilibrium pressure. Then the valve opens and the mercury enters the cell confining the system. The required temperature In the thermostat is set and contents of the cell is mixed with the agitator driven by a ring magnet until l equilibrium is established. It requires about 2 – 3 minutes. After measurement at a given pressure is made the system is compressed and experiment is conducted at a new pressure value. In such a way one receives information about the behavior of the liquid phase at the fixed temperature in a wide range of pressures. Then the thermostat temperature is changed and experiment is carried out again. Thus, at a single cell load one can obtain information in a wide range of temperatures and pressures.
Conclusion
The solubility of carbon dioxide in the organic absorbent containing 47.1 mol%. DEG and 52.9 mol%. NMP in the temperature range of 0-50°C and pressures up to 9 MPa has been investigated. Molar heats of dissolution for carbon dioxide in the absorbent of the composition mentioned were determined. Results of the present research demonstrate high absorbent efficiency relatively to carbon dioxide. Previous investigations showed high absorption capacity of the absorbent to hydrogen sulfide and water vapor. Thus, the investigated absorbent is suitable for complex purification of natural and process gases from the most common contaminants.
Based on the foregoing, it is appropriate to develop a highly efficient method for purification of gas mixtures from carbon dioxide, based on the phenomenon of physical absorption of it by the absorbent.
Further research is focused on the following aspects:
- Solubility determination of carbon dioxide in the organic absorbents of various compositions.
- Construction of the liquid-vapor diagram for the system carbon dioxide – DEG – NMP.
- The development of the technological scheme for purification of gases from carbon dioxide by organic absorbent.
This master's work is not completed yet. Final completion: December 2013. The full text of the work and materials on the topic can be obtained from the author or his adviser after this date.
References
- Тер-Саркисов Р.М. Разработка месторождений природных газов. – М.: ОАО Издательство
Недра
, 1999. – 659 с.: ил. - Семенова Т.А., Лейтес И.Л. и др. Очистка технологических газов. – М.:
Химия
, 1969. – 392 с. - High-pressure fluid-phase equilibria: Experimental methods and systems investigated (2005–2008) / Jose M.S. Fonseca, Ralf Dohrn, Stephanie Peper // Fluid Phase Equilibria 300 (2011) 1–69.
- Hochgesand G. Rectisol and Purisol / Hochgesand G. // Ind. and Eng. Chem. – 1970. –V.62. – No 7. – P. 37–43.
- Коуль А.Л., Ризенфельд Ф.С. Очистка газа – М.: Недра, 1968. – 411 с.
- Краткая химическая энциклопедия. ред. кол. И.Л. Кнунянц (отв. ред.) и др., – М.:
Большая российская энциклопедия
, 1992. – Т. 3, Мед – Полимерные. – 639 с. - Краткая химическая энциклопедия. ред. кол. И.Л. Кнунянц (отв. ред.) и др., – М.:
Большая российская энциклопедия
, 1990. – Т. 2, Даффа – Мед. – 671 с. - Мурин В.И. Технология переработки природного газа и конденсата. Т 38, Ч.1 – М.: ООО
Недра-Бизнесцентр
, 2002 – 517 с. - Равновесие жидкость – пар в системе диоксид углерода – диэтиленгликоль / Матвиенко В.Г., Нифантова Л.С. // Наукові праці Донецького національного технічного університету. Серія: хімія і хімічна технологія. Випуск 144 (12). Донецьк - 2009. С. 67 – 74.
- High-pressure fluid-phase equilibria: Experimental methods and systems investigated (2000–2004) / Ralf Dohrn, Stephanie Peper, Jose M.S. Fonseca // Fluid Phase Equilibria 288 (2010) 1–54.
- High-pressure fluid phase equilibria: Experimental methods and systems investigated (1994–1999) / M. Christov, R. Dohrn // Fluid Phase Equilibria 202 (2002) 153–218.
- Solubility of H2S and CO2 in diethylene glycol at elevated pressures / F.Y. Jou, F.D. Otto, A.E. Mather // Fluid Phase Equilibr. 175 (2000) 53–61.
- Solubility of carbon dioxide in dimethylsulfoxide and N-methyl-2-pyrrolidone at elevated pressure / R. Rajasingam, L. Lioe, Q.T. Pham, F.P. Lucien // J. Supercrit. Fluids 31 (2004) 227–234.
- Experimental setup to measure critical properties of pure and binary mixtures and their densities at different pressures and temperatures. Determination of the precision and uncertainty in the results / Laura Gil, Santos F. Otin, Jose Munoz Embid, M. Asuncion Gallardo, Sofia Blanco, Manuela Artal, Inmaculada Velasco // J. of Supercritical Fluids 44 (2008) 123–138.
- High-Pressure Vapor-Liquid Equilbria of Some Carbon Dioxide + Organic Binary Systems / Michael J. Lazzaroni, David Bush, James S. Brown, and Charles A. Eckert // J. Chem. Eng. Data 2005, 50, 60-65.
- Флюидные фазовые равновесия в системе диоксид углерода-диэтиленгликоль-γ-бутиролактон при повышенных давлениях / В.Г. Матвиенко, Л.С. Нифантова, Н.Ю. Траховцов, В.В. Кулик // Наукові праці Донецького національного технічного університету. Серія: хімія і хімічна технологія. Випуск 19 (199). Донецьк – 2012. С. 40.
- Циклис Д.С. Техника физико-химических исследований при высоких и сверхвысоких давлениях – М.: Химия, 1976. – 432 с.
- Isothermal phase equilibria in binary mixtures consisting of (methanol + hydrogen or nitrogen or methane or carbon monoxide or carbon dioxide) / E. Brunner, W. Hultenschmidt, and G. Schlichtharle // J. Chem. Thermodinamics 1987, 19, 213-291.
- Растворимость аргона в бензоле и гексане при повышенных давлениях / Матвиенко В.Г., Ярым-Агаев Н.Л., Грознер Ю.Л. // ЖПХ. – 1995. – Т.68. – № 2. – С. 198–202.
- Алтунин В.В. Теплофизические свойства двуокиси углерода – М.: Издательство стандартов, 1975. – 546 с.