Abstract
Contents
- Introduction
- 1. Relevance of theme
- 2. Experimental
- 2.1 Synthesis of oxalate precursor of lead zirconate-titanate
- 2.2 Synthesis of nanocrystalline powders of lead zirconate-titanate
- 2.3 Instrumental methods of research
- 3. Preparation of consolidated nanostructured piezoceramics of lead zirconate-titanate
- 3.1 The microstructure of nanostructural ceramics
- 3.2 Electrophysical properties of lead zirconate-titanate
- Conclusion
- References
Introduction
Due to its remarkable electrophysical properties solid solutions of lead zirconate-titanate (PZT) [1] for decades constitute the most widely used basic ferroelectric and piezoelectric materials. Below the ferroelectric Curie temperature ТС they have noncentrosymmetric perovskite structure and many properties exhibit a maximum at the morphotropic phase boundary (in the vicinity of Pb(Ti0,48Zr0,52)O3, where the changing ratio Ti/Zr transition from tetragonal to rhombohedral distortion of the unit cell.
Currently, the potential to improve the properties of ferroelectric and piezoelectric ceramics PZT due to complications and optimal choice of the sintering conditions of samples [2] is almost exhausted. Attracted the attention of researchers prospects of creating nanocrystalline materials, bulk and membranous consolidated nanostructured products [3]. The traditional method of fabrication of piezoelectric ceramics PZT is a ceramic synthesis [4]. For nanocrystalline oxide perovskite family used high-energy mechanical activation [5], laser deposition [6], different methods of solution chemistry [7–11]: co-precipitation, hydrothermal synthesis, sol-gel process, thermolysis and combustion precursors [12–15], etc.
At the nanoscale, a more abrupt than for larger particles, the dependence of the properties of the crystallite size. Ferroelectric Curie temperature ТС unconsolidated nanocrystalline BaTiO3 powders and other perovskite oxides decreases with decreasing crystallite size, indicating suppression of the ferroelectric state.
Much less work devoted to studying the properties of consolidated nanostructured ceramics PZT. The samples of Pb(Ti0,47Zr0,53)O3, prepared by the sol-gel method and consolidated by sintering, marked increase in the relative permittivity ε/ε0 with crystallite size decreases from 150 nm to 40 [5].
The purpose of the master’s work is obtaining a consolidated nanostructured piezoceramics of lead zirconate-titanate (PZT) and the analysis of its properties.
The objective of the master’s work is studying the conditions and kinetics of sintering samples, recrystallization grain growth and electrophysical properties of the resulting consolidated nanostructured PZT piezoelectric ceramics.
1. Relevance of theme
Investigation of the mechanisms and kinetics of synthesis of nanocrystalline powders perovskites is actual scientific problem. Despite the fact that, to date, developed a large number of preparative methods for nanomaterials, the problem of synthesis of nanostructured materials based perovskites have not been fully resolved.
In the master’s work samples of compact ceramic lead zirconate-titanate (PZT) sintered nanocrystalline (dav = 25 nm) powder Pb(Ti0,48Zr0,52)O3, synthesized by thermal decomposition of oxalate precursor. Conditions of formations nanopowders studied the kinetics of sintering and growth of nanoscale crystallites and larger grains formations formed during consolidation are found.
Consolidated nanopowders of PZT ceramics is sintered at temperatures below 300–350 °C, and has a high (25–45 %), the dielectric and piezoelectric properties as compared with the samples obtained by traditional ceramic technology. Duplex formed during sintering ceramics grain structure: nanosized crystallites are separated by low-angle boundaries and genetically related to the original nanocrystalline powder particles and separated by boundaries of large-larger microcrystalline grains.
Thus, the obtaining of nanocrystalline ceramics of PZT powder controls the size of the crystallites in the nanometer range and thereby provides the nanostructured nature of the consolidated material.
2. Experimental
2.1 Synthesis of oxalate precursor of lead zirconate-titanate
The following starting reagent-grade materials were used: titanium tetrachloride TiCl4; zirconium oxychloride ZrOCl2·8H2O, Lead nitrate Pb(NO3)2; Ammonia NH3 (25 % aqueous solution); oxalic acid H2C2O4·2H2O; Bidistillat H2O.
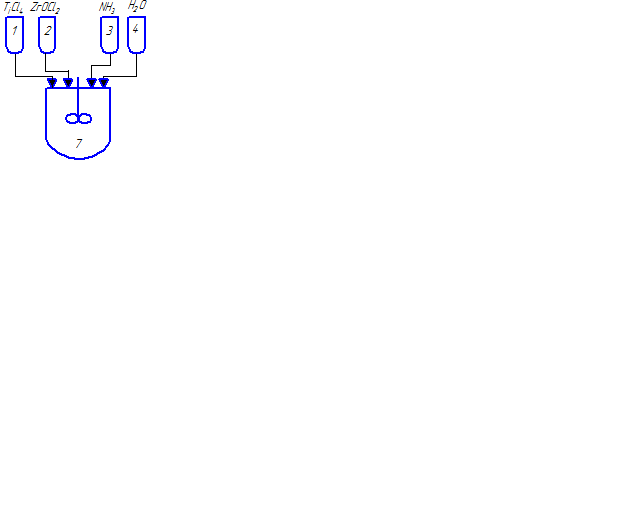
Fig. 2.1 – The technological scheme for the synthesis of nanocrystalline lead zirconate titanate powders
(animation: 7 frames, 8 cycles of repetition, 48 kilobytes)
(1–6 – tanks for initial reactants; 7–9 – stirred-tank reactors; 10 – vacuum drum filter; 11, 12 – pumps; 13 – drier; 14 – ball mill; 15 – press; 16 – furnace)
2.2 Synthesis of nanocrystalline powders of lead zirconate-titanate
Powdered sample of oxalate precursor was placed in the form of layer thickness of 4 mm in alundum crucible and placed in an oven with silit heaters KO-14. The thermal treatment included heating at 15 °C/min to 370 °C, isothermal exposure at 370 °C for 45 min then rapid heating to a temperature high-gradient field from 370 to 750 °C, holding 45 min at a maximum temperature of 750 °C and then cooling with the furnace.
Nanocrystalline PZT powder obtained by thermal decomposition of the synthesized precursor. It is known that in the temperature interval polythermal mode and the output of the fine perovskite phase is largely dependent on the heating rate [16].
2.3 Instrumental methods of research
Study of the processes of synthesis and consolidation of nanopowder PZT performed methods: X-ray phase analysis (XRD), transmission (TEM) and scanning (SEM) electron microscopy, thermogravimetry, dilatometry. Electrophysical properties were measured by the resonance-antiresonance.
X-ray diffraction studies of samples were performed on a DRON-3 diffractometer in the filtered Cu-Kα radiation. Identification of diffractograms
was done by means of ASTM catalog – American Society for Testing Materials
. The average crystallite size dc (coherent scattering – CSR) was calculated by the Scherrer equation:
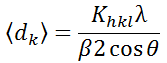
where Khkl – Scherrer constant; λ – X-ray wavelength; β – true physical broadening of the X-ray peak at half its height; θ – angle of reflection.
The morphology and particle size of the powders were investigated by transmission electron microscope (TEM) JEM 200A (JEOL), and scanning electron microscope (SEM) JEOL TSM-T30. The specific surface area was measured by multi-layered powders argon adsorption (BET) on the device SoftSorbi-II.
Dielectric properties (capacity, conductivity and dielectric loss) of powders and ceramic samples were studied on the E 7–8 type LCR meter on frequency of 1 kHz and an output voltage of less than 1 B. The piezoelectric properties were measured by the resonance-antiresonance method on samples in the form of discs Ø 20 x 1 mm at a frequency of 1 kHz. Samples was polarized in the liquid polyethylsiloxane in the field of 4 kV/mm at 120–150 °C for 30 min, then cooled in field to room temperature.
3. Preparation of consolidated nanostructured piezoceramics of lead zirconate-titanate
From the synthesized powder were formed and sintered ceramic products in the form of discs Ø 10 x 1–1,5 mm. High dispersion, uniformity in size and large interparticle adhesion pose significant obstacles to the formation of nanocrystalline samples. As seen from the compression curves (Fig. 3.1), the total porosity θ of nanocrystalline powder, that was compacted without a binder under a pressure of 300 MPa, is greater than 45 %, and these compacts disintegrate easily. To obtain satisfactory results at the stage of forming needed liquid surfactant-based binders that provide sliding and spinning nanocrystals, and increasing the compaction pressure up to 600 MPa. Best results were obtained using a complex binder based solutions dibutyl sebacate (DBS) and polyvinyl butyral (PVB) in acetone.
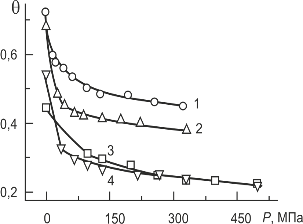
Fig. 3.1 – Compression curves of nano- (1,2) and micro- (3.4) crystalline powders of PZT; 1,3 – without a binder, 2,4 – with a binder DBS + PVB.
Dilatometric shrinkage curves compacts of synthesized powders (fig. 3.2) show significant reduction of sintering temperature of nanocrystalline powder of PZT as compared to the conventional technology. In polythermal mode at a heating rate of 10 °C/min shrinkage of compacts nanocrystalline PZT is completed to 900 °C that is at 300–350 °C lower than for samples of ceramic technology.
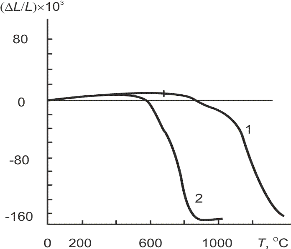
Fig. 3.2 – Dilatometric curves during sintering PZT samples: 1 – traditional ceramic method; 2 – nanocrystalline powder of oxalate precursor. The heating rate is 10 °C/min.
3.1 The microstructure of nanostructural ceramics
Electron microscopy (SEM) studies show (Fig. 3.3) in the temperature range of sintering these processes are accelerated and lead to the formation and further growth of the enlarged, microcrystalline grain structure. The microcrystalline grains do not appear as a result of normal growth of output nanocrystalline particles, they do appear as a result of their adaptation and integration.
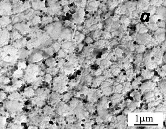
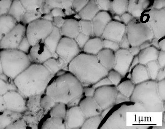
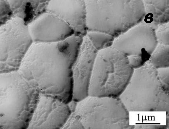
Fig. 3.3 – Formation and growth of microcrystalline grains during sintering of nanocrystalline PZT at 850 °C. Exposure time, min: a – 30; b – 60; c – 180.
Individual nanosized crystallites (CSR) within the existing grains do not completely lose their individuality and also tend to increase with increasing temperature. However, the kinetics of growth of crystallites and the grain is significantly different (Fig. 3.4).
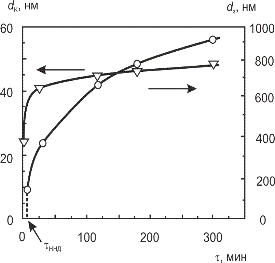
Fig. 3.4 – Comparison of the kinetics of grain growth dg and crystallites dc during sintering of nanocrystalline PZT at 850 °C.
At the initial stage of sintering at 850 °C the average crystallite size (dc) rapidly over 30 min changes from an initial size of 25 to 45 nm, and then slows dramatically during further exposure. In contrast, the growth of micron grains (dg) with time is not braked so sharply.
It follows that the real advantage of PZT nanocrystalline powders ceramic is controlled by providing nanometer-sized crystallites (CSR), and thus the nature of the consolidated nanostructured material.
Fig. 3.5 compared characteristic fraktograms – SEM pictures of chips of PZT ceramics sintered from nanocrystalline powder at 850 °C for 1 hr (dg = 0.65 µm, dc = 45 nm) and PZT ceramics obtained from the traditional method of microcrystalline powder sintering at 1250 °C for 1 hr (dg = 7 µm, dc = 200 nm). Pictures demonstrate the advantages of nanostructured ceramics. PZT ceramics obtained by the conventional method (Fig. 3.5 b), is characterized by a large spread of grain sizes, and contains visible intragranular and intergranular pores.
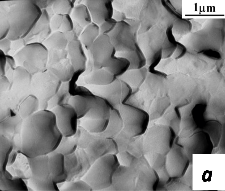
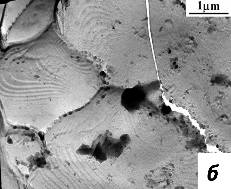
Fig. 4.5 – The grain microstructure of sintered ceramics PZT: a – ceramics, consolidated from nanocrystalline powder; b – the traditional method of synthesis and sintering.
PZT ceramics, sintered from nanocrystalline powder (Fig. 3.5 a) has a submicron grain structure and more uniform grain size distribution, dense grain boundaries, in which practically no pores.
3.2 Electrophysical properties of lead zirconate-titanate
As seen from Table. 3.6, the electrophysical properties of ceramics Pb(Zr0.52Ti0.48)O3, consolidated from nanocrystalline powders (nc), is significantly higher than that of ceramics produced by the conventional method (cm) on d31 and d33 piezoelectric coefficients by 25–30 %, relative to permittivity ε33/ε0 – by 45 %. Also improved electromechanical coupling coefficient Кр, mechanical quality factor Qm, dielectric loss tg δ.
Table 3.6 – Electrophysical properties of PZT piezoelectric ceramics obtained from nanocrystalline powder (nc) and by conventional method (cm).
Samples and microstructure parameters |
ε33/ε0 | tg δ | Кр | Qm | d31, pC/N |
d33, pC/N |
Tc, °C |
---|---|---|---|---|---|---|---|
Pb(Zr0,52Ti0,48)O3, (nc) (dg = 0,65 µm, dc = 45 nm) |
1100 ± 60 | 0,0030 | 0,54 | 650 | 120 ± 5 | 270 ± 10 | 385 |
Pb(Zr0,52Ti0,48)O3, (cm) (dg = 7 µm, dc = 200 nm) |
760 ± 70 | 0,0040 | 0,52 | 500 | 90 ± 7 | 220 ± 15 | 385 |
High dielectric and piezoelectric properties of the PZT piezoelectric ceramics produced from nanocrystalline powders to confirm that the nano-sized crystallites within the same microcrystal grains are bound – they do not interfere with low angle boundaries combinations of atomic vibrations in an ensemble of oscillators within a single ferroelectric domain.
This differs from the consolidated ceramic mixture free nanocrystallites, wherein the particle size reduction results in a reduction of the Curie temperature of the ferroelectric properties and suppression. Simultaneously, local mechanical stresses in the developed interfaces nanocrystals, in particular, on the dislocation and disclination in PZT can lead to the formation pseudo morphotropic areas, facilitate the movement of domain walls, the reorientation of the polarization and increase the associated electrophysical properties. In PZT ceramics consolidated from nanocrystalline powder such an interface are more developed than in samples obtained by traditional technology.
Conclusion
Compact ceramic samples of lead zirconate titanate (PZT) prepared by nanocrystalline powder Pb(Zr0.52Ti0.48)O3 consolidation (dav = 25 nm) by sintering at temperatures lower by 300–350 °C than for traditional ceramic technology. For formation of nanopowders has been matched composition of liquid binders based surfactants, providing sliding and spinning nanocrystallites in the compacts. The kinetics of sintering and grain growth model corresponds seal due to bulk diffusion in transitional periods and the final sintering, while grain growth under the cubic-law.
Consolidation of nanocrystalline powder during sintering leads to a much larger, micrometer grain formations. The latter are formed not as a result of normal diffusive growth of nanocrystalline particles, they are formed by combining many (103–104) crystallites, which under the influence of high surface energy correlated changed their orientation by slips and spins. As a result, creates a two-tier ceramic grain structure: nano-sized crystallites are separated by low-angle boundaries and genetically related to the nanocrystalline powder particles and crystallites, that separated by boundaries of large-microcrystalline grains. Obtaining of nanocrystalline PZT ceramics powder controls the size of the crystallites in the nanometer range and thereby provides the nanostructured nature of the consolidated material.
Quantitative differences in crystallite size ratio (CSR) and grain, separated by large-angle boundaries, lead to higher dielectric and piezoelectric properties of the PZT piezoelectric ceramics sintered from nanocrystalline powders, compared with the ceramics obtained by conventional method.
References
- Jaffe B. Piezoelectric properties of lead zirconate – lead titanate solid-solution ceramics / B. Jaffe, R. S. Roth, and S. Marzullo // J. Appl. Phys. – 1954. Vol. – 25, No. 6. – P. 809–810.
- Приседский В. В. Нестехиометрические сегнетоэлектрики АIIВIVО3. – Донецк: Ноулидж, 2011. – 267 с.
- Глинчук М. Д. Наноферроики / М. Д. Глинчук, А. В. Рагуля. – Киев: Наука, 2009. – 275с.
- Matsuo Y. Formation of lead zirconate – lead titanate solid solutions./ Y. Matsuo, H. Sasaki // J. Am. Ceram. Soc. – 1965. – Vol. 48. – P. 289–291.
- Praveenkumar B. Size effect studies on nanocrystalline Pb(Zr0.53Ti0.47)O3 synthesized by mechanical activation route / B. Praveenkumar, G. Sreenivasalu, H. H. Kumar, D. K. Kharat, M. Balasubramanian, B. S. Murty // Mater. Chem. Phys. – 2009. – Vol. 117. – P. 338–342.
- Craciun F. Pulsed laser deposition of annocrystalline lead zirconate titanate thin films / F. Craciun, M. Dinescu, P. Verardi, C. Galassi // Nanotechnology. – 1999. – Vol. 10. – P. 81–85.
- Q. F. Zhou, Nanocrystalline powders and fibers of lead zirconate titanate prepared by the sol-gel process / Q. F. Zhou, H. L. W. Chan, C. L. Choy // J. Mater. Process. Technol. – 1997. – Vol. 63. – P. 281–285.
- Y. Faheem, M. Shoaib Sol-gel processing and characterization of phase-pure lead zirconate titanate nano-powders // J. Am. Ceram. Soc. – 2006. – V. 89. – No. 6. – P.2034–2037.
- Meng J. F. Raman phonon modes and ferroelectric phase transitions in nanocrystalline lead zirconate titanate / Q. F. Zhou, H. L. W. Chan, C. L. Choy // Phys. Stat. Sol. (a) – 1997. – Vol. 164. – P. 851–862.
- Garnweitner G. Nonaqueous synthesis of amorphous powder precursors for nanocrystalline PbTiO3, Pb(Zr,Ti)O3, and PbZrO3 / G. Garnweitner, J. Hentschel, M. Antonietti, M. Niederberger // Chem. Mater. – 2005. – Vol. 17. – P. 4594–4599.
- Zhu W. Low temperature processing of nanocrystalline lead zirconate titanate (PZT) thick films and ceramics by a modified sol-gel route / W. Zhu, Z. Wang, C. Zhao, O. K. Tan, H. H. Hng // Jpn. J. Appl. Phys. – 2002. – Vol. 41. – P. 6969–6975.
- Banerjee A. Free-standing lead zirconate titanate nanoparticles: low-temperature synthesis and densification / A. Banerjee, S. Bose // Chem. Mater. – 2004. – Vol. 16. – P. 5610–5615.
- Roy S. Metastable face-centered cubic lead zirconate titanate (PZT) and lead lanthanum zirconate titanate (PLZT) nanocrystals synthesized by auto-ignition of metal-polymer gel / S. Roy, S. Bysakh, J. Subrahmanyam // J. Mater. Res. – 2008. – Vol. 23, No. 3. – P. 719–724.
- Dash M. S. Study on phase formation and sintering kinetics of BaTi0.6Zr0.4O3 powder synthesized through modified chemical route / M. S. Dash, J. Bera, S. Ghosh // Alloys and Compounds. – 2007. – Vol. 430. – P. 212–216.
- Das R. A. Low-temperature preparation of nanocrystalline lead zirconate titanate and lead lanthanum zirconate titanate powders using triethanolamine / R. A. Das, A. Pathak, P. Pramanik // J. Am. Ceram. Soc. – 1998. – Vol. 81, No. 12. – P. 3357–3360.
- Рагуля А. В. Синтез и спекание нанокристаллического порошка титаната бария в неизотермических условиях. IV. Электронно-микроскопическое исследование эволюции морфологии порошков титаната бария / А. В. Рагуля, О. О. Васылькив, В. В. Скороход, Н. В. Даниленко // Порошковая металлургия. – 1998. – № 3/4. – С. 12–20.